
How are megakaryocytes produced?
Megakaryocytes, the precursor of platelets, provide a constant source of platelets to the blood system and are themselves produced through a process called megakaryopoiesis. As with RBCs, MKs are generated through the initial differentiation of HSCs into CMPs. Progressive commitment of CMPs to the megakaryocyte lineage is principally regulated by thrombopoietin (TPO). The maturation of megakaryocyte involves an increase in expression of the cell surface markers, GPIIb/IIIa (also known as CD41 or αडडb/βडडड integrin receptor) and GPIb/GPIX/GPV receptors and a substantial increase in cell mass (50 to 100μm in diameter), which results in cytosolic accumulation of α granules, dense bodies, and platelet-associated proteins like von Willebrand factor (vWF) and platelet factor-4 [39,40]. Several rounds of endomitosis lead nuclear polyploidization and cells with up to 128N in DNA content [41,42]. Once polyploid MKs are produced, cellular processes on the MK body called ‘proplatelets’ begin to appear, with their eventual fragmentation and release resulting in the generation of platelets. On a molecular level, thrombopoiesis is a highly coordinate process, with sophisticated reorganization of membrane and microtubules and precise distributions of granules and organelles [43]. Despite the mechanism of platelet generation from MKs is not completely understood, it appears to be extremely efficient in vivo, with 2,000–10,000 platelets being produced per MK [44,45].
Where are megakaryocytes located?
Megakaryocytes are multilobulated, platelet-producing giant cells that lie against the outside of vascular sinuses in bone marrow (see Thrombopoiesis section in Chapter 3 for more details). Cytoplasmic processes of mature megakaryocytes extend into the sinus lumen, where they develop into proplatelets and subsequently individual platelets.
What are the characteristics of megakaryocytes?
Distinctive Features: Megakaryocytes are bone marrow platelet progenitors, which undergo endomitosis rather than mitosis and cell division. Megakaryocytes are extremely large cells (generally 50 to 150 µm), which have a single nucleus with multiple lobes (2–16).
What is dysmegakaryocytopoiesis?
Dysmegakaryocytopoiesis refers to the presence of maturational and/or morphologic abnormalities in megakaryocytic cells. Apparent maturational arrests with early stages (e.g., promegakaryocytes) predominating may not be reflective of a dysplastic process, but rather may result from immune-mediated reactions against megakaryocyte antigens (see Fig. 9-46, A ). 220 Dysplastic abnormalities that may be present in bone marrow include asynchronous maturation resulting in the formation of dwarf granular megakaryocytes with single or multiple nuclei ( Fig. 9-50, A-C) and large megakaryocytes with nuclear abnormalities including hypolobulation, hyperlobulation, or multiple round nuclei ( Fig. 9-51, A,B ). 39 Megakaryocytic dysplasia may occur in association with IMT and/or IMHA, 502,518 lymphoma, 502 and following drug administration, 276,532 but it most frequently occurs in MDS and AML. * Asynchronous maturation and anisokaryosis of megakaryocytes have been reported in a Cavalier King Charles spaniel with inherited macrothrombocytopenia. 518 Chapter 7 offers information about this disorder.
What factors initiate autoantibody production?
The factors that initiate autoantibody production are unknown. Here, glycoprotein IIb/IIIa is recognized by autoantibody, whereas antibodies that recognize the glycoprotein Ib/IX complex have not been generated at this stage (1). Antibody-coated platelets bind to antigen-presenting cells (macrophages or dendritic cells) through Fcγ receptors and are then internalized and degraded (2). Antigen-presenting cells not only degrade glycoprotein IIb/IIIa thereby amplifying the initial immune response, but also may generate cryptic epitopes from other platelet glycoproteins. Activated antigen-presenting cells express these novel peptides on the cell surface along with costimulatory help and the relevant cytokines that facilitate the proliferation of the initiating CD4-positive T cell clones (3). B cell immunoglobulin receptors that recognize additional platelet antigens are thereby also induced to proliferate and synthesize anti-glycoprotein Ib/IX antibodies in addition to amplifying the production of anti-glycoprotein IIb/IIIa antibodies (4). Antiplatelet autoantibodies may also induce decreased megakaryocyte production and suppressed megakaryocyte maturation, which is compounded by an insufficient level of TPO (5). Finally, cytotoxic T cells may exert cell-mediated lysis on megakaryocytes, as well as on platelets (6).
What is dwarf megakaryocyte?
Dwarf megakaryocytes are smaller than normal mature megakaryocytes and have decreased nuclear ploidy, but their cytoplasm generally contains granules and appears similar to that of blood platelets ( Fig. 2-35 ). Dwarf megakaryocytes are common in the bone marrow of animals with myeloid neoplasms but are only rarely seen in blood.
Where are platelets formed in the lung?
3 Platelet Formation in the Lung#N#Megakaryocytes have been identified in intravascular sites within the lung, leading to a theory that platelets are formed from their parent cell predominantly in the pulmonary circulation.87,107,110–115 Aschoff 116 first described pulmonary megakaryocytes in 1893 and suggested that they originated in the marrow, migrated into the bloodstream, and—because of their massive size—were lodged in the capillary bed of the lung, where they released platelets. This mechanism requires the migration of megakaryocytes from the bone marrow into the circulation. Although the size of megakaryocytes would seem limiting, the transmigration of entire megakaryocytes through endothelial apertures of approximately 3 to 6 μm in diameter into the circulation has been recorded in electron micrographs and by living microscopy of rabbit bone marrow. 117,118 Megakaryocytes express the chemokine receptor CXCR4 and can respond to the CXCR4 ligand SDF-1 in chemotaxis assays. 119 However, both mature megakaryocytes and platelets are nonresponsive to SDF-1, suggesting that the CXCR4 signaling pathway may be turned off during late stages of megakaryocyte development, which may provide a simple mechanism for retaining immature megakaryocytes in the marrow and permitting mature megakaryocytes to enter the circulation, where they can liberate platelets. 120,121 Megakaryocytes are remarkably abundant in the lung and the pulmonary circulation, and some have estimated that 250,000 megakaryocytes reach the lung every hour. In addition, platelet counts are higher in the pulmonary vein than in the pulmonary artery, providing further evidence that the pulmonary bed contributes to platelet formation. 107,112,114 In humans, megakaryocytes are 10 times more concentrated in pulmonary arterial blood than in blood obtained from the aorta. 122 Kaufman and colleagues 86 investigated the possibility of platelet formation in the lung by rearranging the pulmonary vessels in a dog such that the blood from the right side of the heart perfused first the right lung and then was directed to the left lung. The majority of megakaryocytes were found in the right lung, suggesting filtration of megakaryocytes by the pulmonary circulation. Despite these observations, the estimated contribution of pulmonary megakaryocytes to total platelet production remains unclear, as values have been estimated from 7 to 100%. 111,123,124 Furthermore, experimental results using accelerated models of thrombopoiesis in mice suggest that the fraction of platelet production occurring in the murine lung is insignificant. Davis and colleagues 125 reported that megakaryocytes and their naked nuclei were rarely observed in lung tissue even after strong stimulation of thrombopoiesis. In theory, proplatelets as well as megakaryocytes may also reach the pulmonary circulation and complete their development into platelets in lung capillaries.
Where do megakaryocytes develop?
Megakaryocytes, like all other cells in the blood, develop from a master stem cell. In adults, these hematopoietic stem cells (HSCs) reside primarily in the bone marrow.1 During mammalian development, stem cells also successively populate the embryonic yolk sac, fetal liver, and spleen. The classical model of hematopoiesis has stated that HSCs are positioned at the top of a developmental hierarchy in which HSCs go through long-term self-renewal as well as give rise to all of the cells of the blood lineage ( Fig. 2.1 ). 2 In this model, self-renewing HSCs gradually lose the ability for self-renewal as they develop into short-term (ST) self-renewing and multipotent progenitors (MPPs), with the first major lineage commitment occurring in MPPs, thus giving rise to progenitors that initiate the myeloid and lymphoid arms of hematopoiesis. 3, 4 Then, bipotent megakaryocyte-erythrocyte (MEP) and granulocyte-macrophage progenitors (GMP) within the myeloid lineage give rise to unipotent progenitors that ultimately develop into all mature progeny. MEPs give rise to erythroid progenitors and unipotent megakaryocytic precursors. 4, 5 Nevertheless, recently this developmental structure has been disputed, with the origin of megakaryocyte precursors being one of the most debated subjects. Studies have suggested that megakaryocytes can be generated from multiple pathways and that some differentiation pathways do not require transit through a requisite multipotent or bipotent MEP stage. 2, 6 These studies suggest that HSCs contain a subset of cells with biased megakaryocyte potential, with megakaryocytes directly arising from HSCs under steady-state and stress conditions.
How do megakaryocytes become polyploid?
Megakaryocytes undergo endomitosis and become polyploid through repeated cycles of DNA replication without cell division. 23, 24, 25 Polyploidization is essential for efficient platelet production. At the end of the proliferation phase, mononuclear megakaryocyte precursors exit the diploid state to differentiate and undergo endomitosis, resulting in a cell that contains multiples of a normal diploid chromosome content (i.e., 4 N, 16 N, 32 N, 64 N). 26 Although the number of endomitotic cycles can range from two to six, the majority of megakaryocytes undergo three endomitotic cycles to attain a DNA content of 16 N. Megakaryocyte polyploidization results in a functional gene amplification, the likely purpose of which is an increase in protein synthesis in parallel with cell enlargement.27 It was initially postulated that polyploidization may result from an absence of mitosis after each round of DNA replication. However, studies of primary megakaryocytes in culture indicate endomitosis does not result from a complete absence of mitosis, but rather a prematurely terminated mitosis. 27, 28, 29 Megakaryocyte progenitors initiate the cycle and undergo a short G1 phase, a typical 6- to 7-h S phase for DNA synthesis, a short G2 phase, followed by endomitosis.30 Megakaryocytes begin the mitotic cycle and proceed from prophase to anaphase A, but do not enter anaphase B, telophase, or undergo cytokinesis. During polyploidization of megakaryocytes, the nuclear envelope breaks down and an abnormal spherical mitotic spindle forms ( Fig. 2.2 ). Each spindle attaches chromosomes that align to a position equidistant from the spindle poles (metaphase). Sister chromatids segregate and begin to move toward their respective poles (anaphase A). However, the spindle poles fail to move apart and do not undergo the separation typically observed during anaphase B. Individual chromatids are not moved to the poles, and subsequently a nuclear envelope reassembles around the entire set of sister chromatids, forming a single, enlarged, but lobed, nucleus with multiple chromosome copies. The cell then skips telophase and cytokinesis to enter G1. This failure to separate sets of daughter chromosomes fully may prevent the formation of a nuclear envelope around each individual set of chromosomes. 28, 29
What is the role of cytoskeleton in platelet formation?
The cytoskeleton of the mature platelet plays a crucial role in maintaining the discoid shape of the resting platelet and is responsible for the shape change associated with platelet activation. This same set of cytoskeletal proteins provides the force to bring about the shape changes associated with megakaryocyte maturation.94 Two cytoskeletal polymer systems exist in megakaryocytes: actin and tubulin. Both of these proteins reversibly polymerize into cytoskeletal filaments. Accumulating evidence supports a model of platelet formation in which microtubules and actin filaments play a crucial role ( Fig. 2.7 ).
What are the secretory granules of megakaryocytes?
Megakaryocyte maturation is characterized by the progressive formation and appearance of a variety of secretory granules. The most abundant are α granules, which contain proteins essential for platelet adhesion during vascular repair (see Chapter 19 ). These granules are typically 200 – 500 nm in diameter and have spherical shapes with a dark central core. They are present in early-stage megakaryocytes and originate from the trans-Golgi network, where their characteristic dark nucleoid core becomes visible within the budding vesicles.56 α Granules acquire their molecular contents from both endogenous protein synthesis and by uptake and packaging of plasma proteins by receptor-mediated endocytosis and pinocytosis.57 Endogenously synthesized proteins such as platelet factor 4, β-thromboglobulin, and vWF are detected in megakaryocytes before endocytosed proteins such as fibrinogen. In addition, synthesized proteins predominate in the juxtanuclear Golgi area, whereas endocytosed proteins are localized in the peripheral regions of the cell.58 It has been well documented that uptake and delivery of fibrinogen to α granules is mediated by integrin αΙIbβ3. 59, 60, 61 Several membrane proteins critical to platelet function are also packaged into α granules, including integrin αIIbβ3, P-selectin (CD62P), and CD36. Although little is known about the intracellular tracking of proteins in megakaryocytes and platelets, experiments using ultrathin cryosectioning and immunoelectron microscopy suggest multivesicular bodies are a crucial intermediate stage in the formation of platelet α granules.62 Multivesicular bodies are prominent in cultured megakaryocytes, but less numerous in bone marrow megakaryocytes. During megakaryocyte development, these large (up to 0.5 μm) multivesicular bodies undergo a gradual transition from granules containing 30- to 70-nm internal vesicles to granules containing predominantly dense material. Internalization kinetics of exogenous bovine-serum albumin-gold particles and of fibrinogen position the multivesicular bodies and α granules sequentially in the endocytic pathway. Multivesicular bodies contain the secretory proteins vWF and β-thromboglobulin, the platelet-specific membrane protein P-selectin, and the lysosomal membrane protein CD63, suggesting they are a precursor organelle for α granules.62 Dense granules (or dense bodies), approximately 250 nm in size, identified in electron micrographs by virtue of their electron-dense cores, contain a variety of hemostatically active substances that are released upon platelet activation, including serotonin, catecholamines, ADP, adenosine 5′-triphosphate (ATP), and calcium. Immunoelectron microscopy studies have also indicated that multivesicular bodies are an intermediary stage of dense granule maturation and constitute a sorting compartment between α granules and dense granules.63
What is the IMS of a megakaryocyte?
One of the most striking features of a mature megakaryocyte is its elaborate IMS ( Fig. 2.3 )—an extensive network of membrane channels composed of flattened cisternae and tubules. The suborganization of the megakaryocyte cytoplasm into membrane-delineated “platelet territories” was first reported by Kautz and De Marsh,45 and a detailed description of these membranes by Yamada 46 soon followed. The IMS is detectable in promegakaryocytes, but becomes most evident in mature megakaryocytes in which it permeates the megakaryocyte cytoplasm, except for a rim of cortical cytoplasm from which it is excluded ( Fig. 2.3 ). It has been proposed that the IMS derives from megakaryocyte plasma membrane in the form of tubular invaginations. 47 The IMS is in contact with the external milieu and can be labeled with extracellular tracers, such as ruthenium red, lanthanum salts, and tannic acid. 47, 48, 49 The exact function of this elaborate smooth membrane system has been hotly debated for many years. Initially, it was postulated to play a central role in platelet formation by defining preformed “platelet territories” within the megakaryocyte cytoplasm (discussed later). However, recent studies more strongly suggest that the IMS functions primarily as a membrane reserve for proplatelet formation and extension. Eckly et al. have begun to shed some light on IMS formation, describing how the IMS forms and matures. To form the IMS, the plasma membrane of megakaryocytes enfolds at specific sites and a perinuclear pre-IMS is generated. 50 Next, the pre-IMS is expanded into its mature form by material added from golgi-derived vesicles and endoplasmic reticulum-mediated lipid transfer. This structural description is in accordance with the studies on platelet glycosyltransferases, which arrive early in the forming IMS and eventually make their way to the megakaryocyte and platelet surfaces.51 Only a small number of proteins have been identified thus far to participate in the IMS formation process based on alterations in its structure in certain KO animals. Gross disruptions of this network are found in megakaryocytes isolated from either filamin a or pacsin2 KO mice, the former of which connects GPIbα to the actin cytoskeleton.52
How do platelets form?
Although it has been universally accepted that platelets derive from megakaryocytes, the mechanisms by which platelets form and release from these precursor cells remain controversial. Throughout the years, several models of platelet production have been proposed. These include (a) platelet budding, (b) cytoplasmic fragmentation via the IMS, and (c) proplatelet formation ( Fig. 2.4 ). Past studies attempting to discriminate between these mechanisms of platelet biogenesis have been hampered by the requirement of sampling bone marrow to obtain megakaryocytes, the relative infrequency of megakaryocytes in the marrow, and the lack of in vitro systems that faithfully reconstitute platelet formation. However, the discovery of thrombopoietin (TPO), a cytokine that binds to the megakaryocyte-specific receptor c-MPL and promotes the growth and development of megakaryocyte precursors (see Chapter 61 ), has led to the emergence of culture systems that recapitulate platelet biogenesis and resulted in a new understanding of the terminal differentiation phase of thrombopoiesis. Several models of platelet biogenesis are discussed next.
What are committed megakaryocyte precursors?
Committed megakaryocyte precursor cells develop from pluripotential hematopoietic progenitors ( Fig. 2.1 ). All hematopoietic progenitors express surface CD34 and CD41, and commitment to the megakaryocyte lineage is indicated by expression of CD61 (integrin β3, GPIIIa) and elevated CD41 (integrin αIIb, GPIIb) levels. From the committed myeloid progenitor cell (colony-forming unit-granulocyteerythroid-macrophage-megakaryocyte [CFU-GEMM]), there is strong evidence for a bipotential progenitor intermediate between the pluripotential stem cell and the committed precursor that can give rise to biclonal colonies composed of megakaryocytic and erythroid cells. 12, 13, 14 Diploid precursors that are committed to the megakaryocyte lineage have traditionally been divided into two colonies based on their functional capacities. 15, 16, 17, 18 The megakaryocyte burst-forming cell is a primitive progenitor that develops from a mixed lineage, bipotential erythroid/megakaryocytic cell. The morphology of the megakaryocyte burst-forming cell does not resemble a mature megakaryocyte, but rather resembles a small lymphocyte. Its high proliferation capacity gives rise to large megakaryocyte colonies. Under appropriate culture conditions, the megakaryocyte burst-forming cell can develop into 40 – 500 megakaryocytes within 1 week. The colony-forming cell is a more mature megakaryocyte progenitor that gives rise to a colony containing 3–50 mature megakaryocytes that vary in their proliferation potential. Megakaryocyte progenitors can be readily identified in bone marrow by immunoperoxidase and AChE labeling. 19, 20, 21 Although both human megakaryocyte colony-forming and burst-forming cells express the CD34 antigen, only colony-forming cells express the HLA-DR antigen.22
Where are megakaryocytes found?
Megakaryocytes are rare myeloid cells (constituting less than 1% of these cells) that reside primarily in the bone marrow (1) but are also found in the lung and peripheral blood. In early development, before the marrow cavities have enlarged sufficiently to support blood cell development, megakaryopoiesis occurs within the fetal liver and yolk sac. Megakaryocytes arise from pluripotent HSCs that develop into 2 types of precursors, burst-forming cells and colony-forming cells, both of which express the CD34 antigen (2). Development of both cell types continues along an increasingly restricted lineage culminating in the formation of megakaryocyte precursors that develop into megakaryocytes (1). Thrombopoietin (TPO), the primary regulator of thrombopoiesis, is currently the only known cytokine required for megakaryocytes to maintain a constant platelet mass (3). TPO is thought to act in conjunction with other factors, including IL-3, IL-6, and IL-11, although these cytokines are not essential for megakaryocyte maturation (4).
How do megakaryocytes produce platelets?
Megakaryocytes tailor their cytoplasm and membrane systems for platelet biogenesis. Before a megakaryocyte has the capacity to release platelets, it enlarges considerably to an approximate diameter of 100 μm and fills with high concentrations of ribosomes that facilitate the production of platelet-specific proteins (5). Cellular enlargement is mediated by multiple rounds of endomitosis, a process that amplifies the DNA by as much as 64-fold (6–9). TPO, which binds to the c-Mpl receptor, promotes megakaryocyte endomitosis. During endomitosis, chromosomes replicate and the nuclear envelope breaks down. Although interconnected mitotic spindles assemble, the normal mitotic cycle is arrested during anaphase B. The spindles fail to separate, and both telophase and cytokinesis are bypassed. Nuclear envelope reformation (10, 11) results in a polyploid, multilobed nucleus with DNA contents ranging from 4N up to 128N within each megakaryocyte (12).
What is the process of proplatelet formation?
Nascent platelets forming at the proplatelet tips must be loaded with their contents of organelles and platelet-specific granules. This process occurs along the shafts of proplatelets, as organelles and granules travel in a discontinuous fashion from cell body to proplatelet. Despite bidirectional movement along shafts, the particles are eventually captured at the proplatelet tip (54). Immunofluorescence and electron microscopy studies indicate that organelles are in direct contact with microtubules (48), and their movement appears to be independent of actin. Of the 2 major microtubule motors, kinesin and dynein, only the plus end–directed kinesin is situated in a pattern similar to that of organelles and granules and is likely responsible for transporting these elements along microtubules (54). It appears that a 2-fold mechanism of organelle and granule movement occurs in platelet assembly. First, organelles and granules travel along microtubules, and second, the microtubules themselves can slide bidirectionally in relation to other motile filaments to indirectly move organelles along proplatelets in a “piggyback” fashion.
How do microtubules reorganize proplatelets?
These microtubules align into bundles and fill the cortex of the first blunt process extended by megakaryocytes, signaling the beginning of proplatelet development. The microtubules merge into thick linear bundles that fill the proplatelet shafts when the proplatelets lengthen and taper. At the free proplatelet end, the microtubule bundles form loops, which reenter the proplatelet shaft. This process gives rise to the bulbous tips of the proplatelets, each measuring 3–5 μm in diameter. These studies also were the first to recognize that the platelet-sized swellings that occur along proplatelet shafts are not nascent platelets but are instead points where the microtubule bundles of the shaft diverge for a short distance and then reconvene to locally thicken the proplatelet shaft. Coiling of microtubules, the signature of circulating platelets, occurs only at the proplatelet ends and not within the platelet-sized swelling positioned along the proplatelet shaft. Therefore, the primary site of platelet assembly is at the end of each proplatelet.
How are platelets formed in megakaryocytes?
The assembly of platelets from megakaryocytes involves an elaborate dance that converts the cytoplasm into 100- to 500-μm-long branched proplatelets on which the individual platelets develop. The proplatelet and platelet formation process generally commences from a single site on the megakaryocyte where 1 or more broad pseudopodia form. Over a period of 4–10 hours, the pseudopodial processes continue to elongate and become tapered into proplatelets with an average diameter of 2–4 μm. Proplatelets are randomly decorated with multiple bulges or swellings, each similar in size to a platelet, which gives them the appearance of beads connected by thin cytoplasmic strings (Figure (Figure1).1). The generation of additional proplatelets continues at or near the original site of proplatelet formation and spreads in a wavelike fashion throughout the remainder of the cell until the megakaryocyte cytoplasm is entirely transformed into an extensive and complex network of interconnected proplatelets (27, 36). The multilobed nucleus of the megakaryocyte cell body is compressed into a central mass with little cytoplasm and is eventually extruded and degraded. Platelet-sized swellings also develop at the proplatelet ends and are the primary sites of platelet assembly and release, as opposed to the swellings along the length of the proplatelet shaft (Figure (Figure1).1). The precise events involved in platelet release from proplatelet ends have not been identified.
What is the role of NF-E2 in platelet biosynthesis?
The transcription factor NF-E2 has been identified as a major regulator of platelet biosynthesis. NF-E2 is a heterodimer of p45 and p18 subunits that assumes the basic leucine zipper motif (36). NF-E2 null mice experience lethal thrombocytopenia and die from hemorrhage since they lack circulating platelets (37). Megakaryocytes from NF-E2–null mice fail to undergo proplatelet formation, although megakaryocyte maturation appears intact (38). Interestingly, megakaryocytes from NF-E2 knockout mice lack β1-tubulin, the major β-tubulin isoform expressed in megakaryocytes and platelets (39). NF-E2 has also been shown to interact with the promoter for Rab27b, a small GTPase identified in platelets. Rab27b expression is high in terminally differentiated megakaryocytes, and its inhibition in megakaryocytes results in attenuated proplatelet production, which suggests a role for Rab27b in proplatelet formation (40). NF-E2 may also affect thromboxane synthase (41) and caspase-12 (42), both of which are reduced in NF-E2–null megakaryocytes.
How are platelets released?
Platelets are formed and released into the bloodstream by precursor cells called megakaryocytes that reside within the bone marrow. The production of platelets by megakaryocytes requires an intricate series of remodeling events that result in the release of thousands of platelets from a single megakaryocyte. Abnormalities in this process can result in clinically significant disorders. Thrombocytopenia (platelet counts less than 150,000/μl) can lead to inadequate clot formation and increased risk of bleeding, while thrombocythemia (platelet counts greater than 600,000/μl) can heighten the risk for thrombotic events, including stroke, peripheral ischemia, and myocardial infarction. This Review will describe the process of platelet assembly in detail and discuss several disorders that affect platelet production.
Where do megakaryocytes reside?
Megakaryocytes reside next to the bone marrow sinuses and project pseudopodia through the sinus walls , releasing platelets directly into the circulation
What is the role of megakaryocytes in platelet production?
Megakaryocytes form demarcation membrane within cytosol, which leads to production of platelets. Maturation is associated with acquisition of surface GP Ib, GP IIb and von Willebrand factor. Four types of cytoplasmic granules are alpha, delta, gamma and peroxisomes.
How big is a megakaryoblast?
Megakaryoblast: variable size (7 - 35 microns); may be designated micromegakaryoblast if < 15 microns; round / ovoid cells with scanty blue agranular cytoplasm that often forms a rim around nucleus and may have a few small budding protrusions at periphery; nuclei are round / oval with coarse granular chromatin, one or more nucleoli
Which type of karyocyte is smaller in fetuses and infants?
Megakaryocytes are smaller in fetuses and infants and show less nuclear segmentation when compared to older children
What are the cells that circulate blood?
Circulating blood platelets are specialized cells that prevent bleeding and minimize blood vessel injury. Large progenitor cells in the bone marrow called megakaryocytes (MKs) are the source of platelets. MKs release platelets through a series of fascinating cell biological events. During maturation, they become polyploid and accumulate massive amounts of protein and membrane. Then, in a cytoskeletal-driven process, they extend long branching processes, designated proplatelets, into sinusoidal blood vessels where they undergo fission to release platelets. Given the need for platelets in many pathological situations, understanding how this process occurs is an active area of research with important clinical applications.
Where are platelets formed?
Platelets are formed from the cytoplasm of megakaryocytes (MKs), their precursor cells, which reside in the bone marrow ( Pease, 1956 ). MKs are the largest (50–100 µm) and also one of the rarest cells in ...
How do MKs become polyploid?
To assemble and release platelets, MKs become polyploid by endomitosis (DNA replication without cell division) and then undergo a maturation process in which the bulk of their cytoplasm is packaged into multiple long processes called proplatelets, and the nucleus is extruded.
Where do polyploid MKs migrate?
Polyploid MKs localize to sinusoidal bone marrow endothelial cells in vivo, where they form proplatelets that migrate through bone marrow endothelial cells , and release platelets directly into the marrow intravascular sinusoidal space ( Tavassoli and Aoki, 1989 ).
Where do MKs come from?
MKs develop from hematopoietic stem cells (HSCs) that reside mainly in the bone marrow but are also present in the yolk sac, fetal liver, and spleen during early development ( Long et al., 1982; Gordon et al., 1990; Ogawa, 1993; Morita et al., 2011 ).
Is MK a polyploid?
It is theorized that MKs are polyploid to produce the large quantities of mRNA and protein necessary to be packaged into platelets while still retaining their ability to perform multiple functions without the stress of mitosis and cytokinesis ( Zimmet and Ravid, 2000 ). Although endomitosis and polyploidization are undoubtedly important for MK cytoplasmic maturation, the relationship between high nuclear DNA content and efficient platelet formation is still highly debated. For example, nicotinamide, a form of vitamin B3, is commonly used to increase the ploidy of human and mouse MKs in culture ( Giammona et al., 2006; Panuganti et al., 2010; Avanzi et al., 2012; Leysi-Derilou et al., 2012 ). Although nicotinamide does increase ploidy, its effect on platelet formation is controversial. Some observed nicotinamide-induced increased proplatelet production in vitro ( Giammona et al., 2006 ), whereas others saw reduced proplatelet formation ( Giammona et al., 2006; Leysi-Derilou et al., 2012 ). Importantly, administration of nicotinamide to mice does not increase their platelet levels ( Konieczna et al., 2013 ). In addition, overexpression of cell cycle regulators, such as cyclin D3, has been shown to enhance MK ploidy in vivo without any concomitant increase in platelet count ( Zimmet et al., 1997 ). As we learn more about the process of endomitosis and how to manipulate it, we will be better able to reveal whether high nuclear content is causative or correlative to platelet formation.
Is the cytoskeleton necessary for proplatelet formation?
Although these studies suggest that regulation of the cytoskeleton is necessary for efficient proplatelet formation, their focus is largely on deficiencies or mutations that diminish proplatelet formation. In the future, it will be important to investigate whether direct manipulation of the cytoskeleton can augment or even initiate the process of proplatelet formation directly.
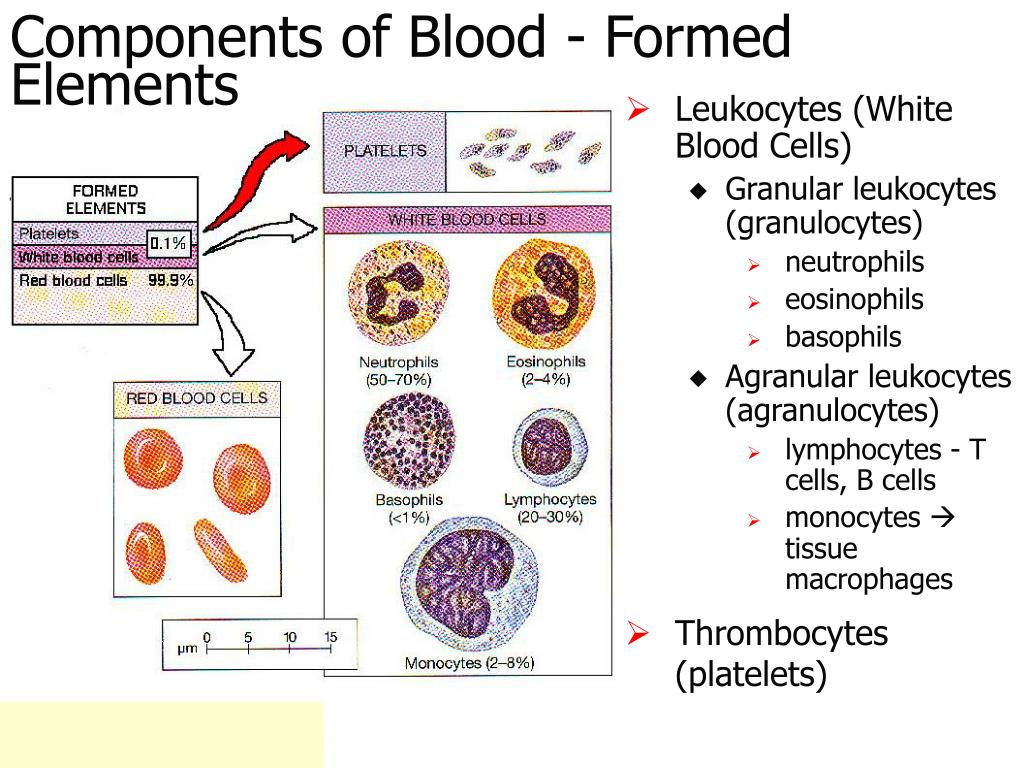