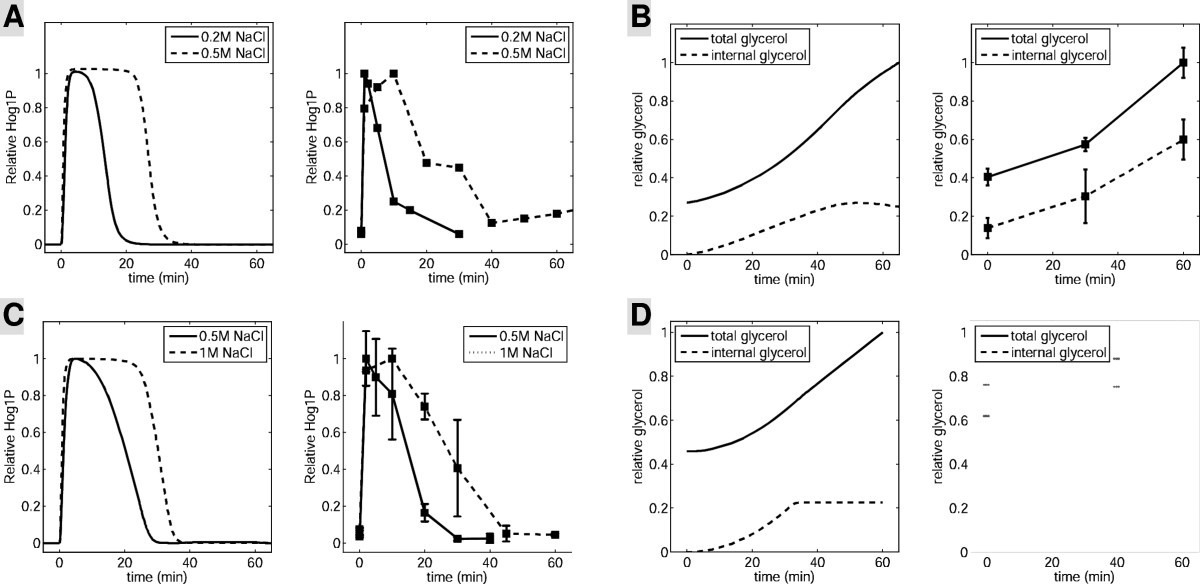
Osmotic shock or osmotic stress is a sudden change in the solute concentration around a cell, causing a rapid change in the movement of water across its cell membrane. Under conditions of high concentrations of either salts, substrates or any solute in the supernatant water is drawn out of the cells through osmosis
Osmosis
Osmosis is the spontaneous net movement of solvent molecules through a semi-permeable membrane into a region of higher solute concentration, in the direction that tends to equalize the solute concentrations on the two sides.
What is the mechanism of osmotic shock?
Osmotic shock. All organisms have mechanisms to respond to osmotic shock, with sensors and signal transduction networks providing information to the cell about the osmolarity of its surroundings; these signals activate responses to deal with extreme conditions. Although single-celled organisms are more vulnerable to osmotic shock,...
What are the biocidal benefits of osmotic shock treatment?
Studies have highlighted the biocidal benefits of osmotic shock treatment for preventing a microbial invasion of filter media and membranes. The explanation for this effect is that the cytoplasm, which is the inner part of the cell composed mainly of water, is surrounded by a semipermeable membrane.
What is the difference between hypotonic and osmotic shock?
In hypotonic solutions water flows into the cell and the cell grows. Osmotic shock or osmotic stress is physiologic dysfunction caused by a sudden change in the solute concentration around a cell, which causes a rapid change in the movement of water across its cell membrane.
Why are cells more resistant to osmotic shock?
Cells that have a cell wall tend to be more resistant to osmotic shock because their cell wall enables them to maintain their shape. Although single-celled organisms are more vulnerable to osmotic shock, since they are directly exposed to their environment, cells in large animals such as mammals still suffer these stresses under some conditions.
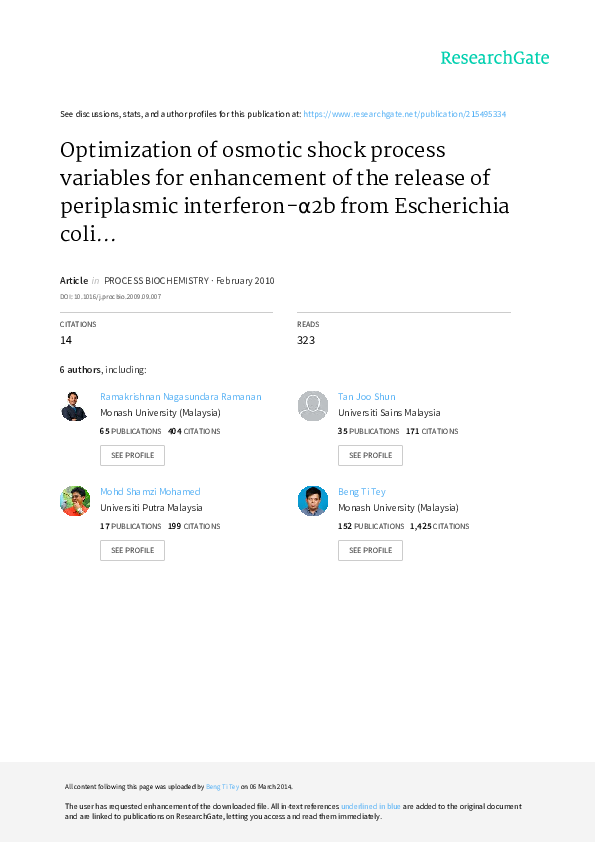
What happens osmotic shock?
Osmotic shock or osmotic stress is physiologic dysfunction caused by a sudden change in the solute concentration around a cell, which causes a rapid change in the movement of water across its cell membrane.
How does osmotic equilibrium work?
By diffusion of water or solutes, osmotic balance ensures that optimal concentrations of electrolytes and non-electrolytes are maintained in cells, body tissues, and in interstitial fluid. Solutes or water move across a semi-permeable membrane, causing solutions on either side of it to equalize in concentration.
How do cells deal with osmotic stress?
The initial cellular response to osmotic stress is the production of osmolytes (e.g., sorbitol and betaine) that stabilize molecular structures and prevent protein misfolding. Prolonged osmotic stress results in delayed cell cycle, DNA damage and apoptosis [98].
What is osmotic shock in RBC?
Osmotic shock triggers eryptosis, a suicidal death of erythrocytes characterized by cell shrinkage, cell membrane blebbing and phosphatidylserine exposure at the cell surface. Phosphatidylserine-exposing erythrocytes are recognized by macrophages, engulfed, degraded and thus cleared from circulating blood.
How is osmotic equilibrium reached?
Water molecules will move from the side of higher water concentration to the side of lower concentration until both solutions are isotonic. At this point, equilibrium is reached.
What causes osmotic pressure?
Osmotic pressure is the pressure caused by water at different concentrations due to the dilution of water by dissolved molecules (solute), notably salts and nutrients.
What controls osmotic pressure in a cell?
Cell vacuoleCell vacuole controls osmotic pressure in a cell.
How does osmotic stress affect the organisms?
Osmotic stress changes cell volume, directly altering macromolecule concentration. This means that osmotic stress is an essential component in the emerging picture of how extracellular physical signals act on the genome.
What happens to red blood cells placed in 0.9% NaCl solution?
If a red blood cell (intracellular concentration of 0.9% salt) is placed into a test tube containing 10% salt solution, what would happen to it? It would fill with water and burst. The red blood cell would shrink as it loses water to the salt solution in the test tube.
What is meant by osmotic?
(oz-MAH-tik) Having to do with osmosis (the passage of a liquid through a membrane from a less concentrated solution to a more concentrated one). This causes the more concentrated solution to become diluted, and makes the concentrations in both solutions more equal.
What is osmotic hemolysis?
Red cell osmotic hemolysis has traditionally been defined by the loss of hemoglobin, in response to reduced osmotic pressure, as measured spectroscopically.
How does osmotic shock work?
Osmotic shock is the technique of weakening cells caused by lysis, which is brought about by increased internal pressure as water rapidly enters cells when cells are subjected to high osmotic pressure followed by sudden dilution (Middelberg, 1995 ). Use of aqueous solution with varying ionic strengths enables the selective extraction of proteins by utilizing the salting-in and/or salting-out phenomenon of food proteins ( Boye and Barbana, 2012 ). Compared to other cell disruption methods, osmotic shocking alone is relatively inefficient in cell disruption with low protein extraction as well as the existence of large variation with species ( Mæhre et al., 2016 ). However, there is always potential for optimization in combination with other methods ( Combined Extraction Process Using Traditional and Emerging Techniques section).
Why is osmotic shock beneficial?
Studies have highlighted the biocidal benefits of osmotic shock treatment for preventing a microbial invasion of filter media and membranes. The explanation for this effect is that the cytoplasm, which is the inner part of the cell composed mainly of water, is surrounded by a semipermeable membrane.
How to minimize osmotic shock?
To minimize osmotic shock differences, a ring of 0.l-µl droplets of the cryoprotectant solution can be placed around a drop containing the crystalon a coverslip. This set up is allowed to equilibrate overnight over a reservoir containing the cryoprotectant solution. The small droplets are then added serially to the crystal drop and equal volumes drawn off. Eventually, larger volumes of the reservoir can be added and the crystal thoroughly washed.
How does osmotic shock affect protein extraction?
Osmotic shock employed on macroalgae improved the extraction of protein. This was found to be directly related to the volume of water, wherein higher volume of osmotic medium relative to immersed/dissolved sample is more useful ( Veide Vilg and Undeland, 2017 ). On the other hand, temperature and incubation time did not significantly affect extraction yield, although longer period of incubation proved to be helpful in obtaining higher concentration of protein from S. vulgare ( Barbarino and Lourenço, 2005 ). Similar trend was observed in Chlorella pyrenoidosa where increasing soaking/incubation time appeared to improve the protein extraction rate significantly. Higher protein extraction in deionized water was suggested to be due to the higher nitrogen solubility whereas increasing salt concentration resulted in reduced solubility ( Kumar et al., 2014 ). In contrast, 0.04% NaCl used in the resuspension of C. vulgaris may have led to osmotic shock but did not result in release of any protein but only small ionic substances. However, protein was released from N. oeloabundans using the same procedure due to osmotic shock ( ’t Lam et al., 2017 ).
What is the cause of osmotic shock?
Osmotic shock. Osmotic shock caused by a sudden change in salt concentration will cause disruption of a number of cell types. Cells are equilibrated to high osmotic pressure (typically 1 M salt solutions). Rapid exposure to low osmotic pressure causes water to quickly enter the cell. This increases the internal pressure ...
How does osmotic regulation occur?
Desiccation, osmotic regulation and osmotic shock occur largely due to the stresses imposed by changes in soil water potential. In simple terms, soil water potential is a measure of the energy required to remove water from the soil. Soil drying is generally a gradual process during which turgor pressure increases as water is removed from the surrounding soil and so the microbial cells release water (generally thought not to contain P) in order to equilibrate this pressure (homeostatic regulation). This means that mortality and cell lysis do not necessarily occur, although if too extreme, desiccation, and mortality will take place (Halverson et al., 2000 ), resulting in the release of microbial P. Similarly anhydrobiosis occurs in some microorganisms in an attempt to avoid damage during freezing. In this process, bulk water within cells is minimized or even eliminated, leaving behind only miniscule amounts of water forming bound or vicinal water shells around macromolecules, resulting in no free water to form damaging ice crystals (see below), but severe desiccation can occur, again potentially leading to P solubilization ( Storey and Storey, 2005 ). Increases in solute concentrations in the soil solution can occur during drying due to the evaporation of water, while during freezing this increase results from the removal of pure water from the soil solution to form ice crystals in the extracellular spaces, rather than in the cell itself. Both processes set up a steep osmotic gradient across cell membranes causing an outflow of water and consequently cell dehydration, elevated intracellular ionic strength and cell volume reduction, all of which cause stress and potential death. The rewetting of dry soils can cause rapid fluctuations in soil matric potential ranging from − 20 MPa to almost 0 ( Evans et al., 1975 ), causing a number of different microbial processes to occur. Rapid increases in turgor pressure can occur, resulting in cell damage, plasmolysis and/or death ( Harris, 1981; Kieft et al., 1987; Salema et al., 1982; Storey and Storey, 2005 ). This results in the release of cell contents of which P is an important component. However, the thicker a microorganisms cell wall, the more likely it is to be able to withstand these pressures ( Harris, 1981 ). Halverson et al. (2000) report that, upon rapid rewetting, cells may actively or passively release intracellular organic and inorganic solutes ( Christian, 1962; Reed et al., 1986 ), as well as catabolize compatible organic solutes to CO 2 or polymerize solutes to reduce osmotic activity ( Berrier et al., 1992; Fierer and Schimel, 2003; Reed and Stewart, 1983 ), thereby avoiding cell lysis and mortality. The chemistry of the rewetting water is also important because if it is not in ionic balance with intracellular solutions this can also increase the likelihood of osmotic shock ( Appel, 1998 ).
How do organisms respond to osmotic shock?
All organisms have mechanisms to respond to osmotic shock, with sensors and signal transduction networks providing information to the cell about the osmolarity of its surroundings; these signals activate responses to deal with extreme conditions.
What is shock caused by?
Shock caused by a sudden change in the solute concentration around a cell. Osmotic shock or osmotic stress is physiologic dysfunction caused by a sudden change in the solute concentration around a cell, which causes a rapid change in the movement of water across its cell membrane.
What is the role of calcium in osmotic stress?
In eukaryotes, calcium acts as one of the primary regulators of osmotic stress. Intracellular calcium levels rise during hypo-osmotic and hyper-osmotic stresses.
Which organisms are more vulnerable to osmotic shock?
Although single-celled organisms are more vulnerable to osmotic shock, since they are directly exposed to their environment, cells in large animals such as mammals still suffer these stresses under some conditions.
Does water shock a cell?
This also inhibits the transport of substrates and cofactors into the cell thus “shocking” the cell. Alternatively, at low concentrations of solutes, water enters the cell in large amounts, causing it to swell and either burst or undergo apoptosis.
What Is Osmotic Shock?
Exposing bacterial cells to high external concentrations of osmolytes (small, soluble compounds that regulate cell volume) retards cell growth and division. This is because the cells lose water to the growth medium through osmosis, resulting in so-called osmotic shock. [1]
Which theory asserts that chemical interactions between the osmolyte and the target protein backbone are energetic?
In short, nobody knows for sure. The theory with the most evidence going for it is the so-called osmophobic theory . [2] . It asserts that chemical interactions between the osmolyte and the target protein backbone are energetically unfavorable.
What is osmotic shock?
Osmotic shock or osmotic stress is a sudden change in the solute concentration around a cell, causing a rapid change in the movement of water across its cell membrane. Under conditions of high concentrations of either salts, substrates or any solute in the supernatant water is drawn out ...
Does water shock a cell?
This also inhibits the transport of substrates and cofactors into the cell thus “shocking” the cell. Alternatively, at low concentrations of solutes, water enters the cell in large amounts, causing it to swell and either burst or undergo apoptosis. Source. Links.
What is the osmotic stress response?
Osmotic stress response is triggered by water and electrolyte imbalance typically caused by dehydration. Given that water comprises approximately 70% of the cell content, osmotic stress can cause critical cell damage. The rapid change in the movement of water and ions across the cell membrane results in cell membrane distortion, protein aggregation and DNA damage. The initial cellular response to osmotic stress is the production of osmolytes (e.g., sorbitol and betaine) that stabilize molecular structures and prevent protein misfolding. Prolonged osmotic stress results in delayed cell cycle, DNA damage and apoptosis [98]. The increased osmotic stress response we have measured in TBI (see Figs 23.1 and 23.10) might be triggered by an abrupt water translocation between intra- and extracellular compartments in the brain driven by the mechanical force during a head injury.
What is the initial cellular response to osmotic stress?
The initial cellular response to osmotic stress is the production of osmolytes (e.g., sorbitol and betaine) that stabilize molecular structures and prevent protein misfolding. Prolonged osmotic stress results in delayed cell cycle, DNA damage and apoptosis [98].
How is HSF induced?
The osmotic stress-induced HSF activation is unique in that: (i) it is induced within minutes after osmotic stress; (ii) it is inducible by both hypo- and hyper-osmolarity; and (iii) it is uncoupled from transcriptional activation. Except for TonEBP, which has yet to be fully identified, HSF is the only known DNA binding protein that is responsive to osmotic stress. Unlike TonEBP, which responds only to hyper-osmotic stress, HSF activation is sensitive to both hypo- and hyper-osmotic stress. In view of its sensitivity to both temperature and osmolarity changes, HSF can be consider not only as a molecular thermostat, but also a molecular osmometer. HSF activation (trimerization and DNA binding) occurs rapidly within minutes under both heat stress and osmotic stress. In contrast, TonEBP activation, as measured by TonE binding activity, occurs more slowly, usually 6–10 h after hyperosmotic stress. The TonE-containing genes are responsible for the slow accumulation of osmolytes in cells for adaptation to prolonged hypertonic conditions. The function of HSF during both hypo- and hyper-osmotic stress is unclear. Whether HSF activation by osmotic stress is related to delayed HSP gene expression or more directly to the protection of chromosomal DNA from nuclease attack at specific sites remains to be studied. One of the major challenges in studying the regulation of the heat shock response is the identification of the intracellular signals that lead to the activation of HSF by a diverse array of inducers, including osmotic stress. If a common signal exists, this signal must be sensitive not only to enthalpy change but also to changes of osmotic pressure in either direction. In this regard, osmotic stress-induced HSF activation also provides a nice model system to study the mechanism of HSF activation.
How does osmotic stress affect DNA?
An important part of the cellular response to osmotic stress is the regulation of the cell cycle, which is closely linked to DNA conformation and DNA activity. For a better understanding of the osmosensory signal transduction networks ...
What does compatible solute do to the cell?
Compatible solutes increase intracellular osmolarity and water again flows into the cell, turgor pressure is restored, and the cells begin to grow again. In addition, osmoprotectants stabilize the native state of globular proteins in the cell.
Does osmotic stress affect cell volume?
Osmotic stress and concomitant cell shrinkage or swelling regulate a wide range of membrane transport proteins by mechanisms that are proposed to include altered membrane thickness, cytoskeletal reorganization, macromolecular crowding, ionic strength, specific ions, and numerous signaling pathways that are sensitive to osmotic perturbations or cellular volume changes (for a review, see Hoffmann, Lambert, & Pedersen, 2009 ). The different NHE isoforms respond differently to osmotic stress. Again, most available data on the mechanisms concern the shrinkage-activation of NHE1, and this is discussed in detail below. Intriguingly, for NHE2, shrinkage-activation ( Kapus et al., 1994 ), shrinkage-inhibition ( Nath et al., 1996 ), and no effect of shrinkage ( Su, Pang, Wakabayashi, & Shigekawa, 2003) have been reported in various cell types, and may point to the importance of non- NHE -intrinsic components regulating its volume sensitivity, some of which may be membrane constituents binding to the C-tail. In PS120 fibroblasts, replacing the first extracellular loop in NHE2 with that of NHE1 caused NHE2 to become shrinkage-activated (which in these cells it is normally not). This was interpreted to reflect the existence of an inhibitory role of an NHE2 -specific part of this loop in shrinkage-activation ( Su et al., 2003 ). In contrast, there is agreement that NHE3 is inhibited by osmotic shrinkage ( Good, Di Mari, & Watts, 2000; Kapus et al., 1994; Nath et al., 1996; Soleimani et al., 1994; Watts & Good, 1999 ), and, conversely, it is found to be activated by cell swelling in most cell types studied ( Alexander et al., 2007; Good et al., 2000; Watts & Good, 1999 ). Interestingly, although NHE3 is generally regulated by insertion in/removal from the plasma membrane ( Section 7 ), studies in MDCK cells showed that swelling-induced NHE3 activation was dependent on membrane deformation but not on insertion of additional NHE3 proteins in the plasma membrane, and, surprisingly, also not on altered interaction of the transporter with the cytoskeleton ( Alexander et al., 2007 ). NHE4 is efficiently shrinkage activated in a manner dependent on the actin cytoskeleton, and has been suggested to play a special role in regulatory volume increase in tissues exposed to very high osmolarity, for example, the kidney medulla ( Bookstein et al., 1996; Bookstein et al., 1994 ). NHE5, which is most closely related to NHE3, is also inhibited by cell shrinkage ( Attaphitaya et al., 2001 ), although to our knowledge, the mechanism remains to be elucidated.
Does NHE1 change Vmax?
In contrast, osmotic activation of NHE1 is not associated with a change in Vmax (e.g., Lacroix et al., 2008; Nath et al., 1996 ), in good agreement with the Phe-gate model proposed above. Lacroix and coworkers also investigated the Monod–Wyman–Changeux model in the context of activation of NHE1 by osmotic cell shrinkage ( Lacroix et al., 2008 ). They demonstrated that a hyperosmotic challenge modifies the L 0 parameter (the allosteric affinity constant) in favor of the activated state. Notably, stimulation of the exchanger with serum shifted the basal equilibrium of NHE1 toward the higher affinity state, but did not prevent osmotic activation of the exchanger ( Lacroix et al., 2008 ).
How does osmotic stress affect cells?
However, in addition to such membrane-mediated regulation of cell signaling, osmotic stress can have physical effects on cells that may extend to the cell nucleus, altering nuclear morphology and genome architecture directly [Albiez et al., 2006; Chalut et al., 2008; Delpire et al., 1985; Finan et al., 2008; Oswald et al., 2006]. Important nuclear processes such as repair, replication and transcription are all spatially organized, thus providing an alternative mechanism by which osmotic stress may influence cell biological function by rearranging the genome without the involvement of a conventional cell signaling pathway. This review summarizes the current state of the literature on the physical response of the cell nucleus to osmotic stress and presents a model of nuclear deformation under altering osmolarity to provide further insights into the mechanisms by which extracellular physical signals can modulate cellular gene expression and function.
How do cells respond to osmotic stress?
A “perfect” osmometer is a theoretical model consisting of a body of solution enclosed by a semi-permeable membrane that allows free passage of water while blocking passage of solute molecules (Fig. 1). If the solute concentration in the bath surrounding the osmometer changes, water flows across the membrane until the concentration inside the osmometer matches the new environmental concentration. Hence, the volume of an osmometer is inversely proportional to the external osmolality, termed the Boyle-van’t Hoff relationship. At room temperature, cells exhibit this passive response [Guilak et al., 2002]. At body temperature, cells typically display some capacity to regulate their volume under osmotic load by pumping ions across the cell membrane so that water is osmotically obliged to cross the membrane in the same direction [Hendil and Hoffmann, 1974]. On a longer timescale, cells may also eject or produce neutral solute molecules such as taurine so that cell volume can be regulated independently of ion concentration [Hallows and Knauf, 1993]. More recently, theoretical models of cells have been proposed that may explain volume regulation from a pure physical standpoint [Albro et al., 2007].
How does osmotic stress affect the actin cytoskeleton?
The shape and mechanics of the nucleus also depend on contractility in the actin cytoskeleton. This dependence is another mechanism by which osmotic stress may act on the nucleus, since hypo-osmotic stress can trigger disassembly of the actin cytoskeleton [Erickson et al., 2003]. Actin filaments assemble into one of two structures: bundled stress fibers or cross-linked filament networks. Actin cross-linking proteins influence the nature of the actin structure assembled. Some, such as filamin, join actin filaments at an angle to form a gel. Others, such as α-actinin, join actin filaments in a parallel orientation to form thick bundles that can exert tension when acted upon by myosin motor proteins. The physical properties of the cell environment affect the actin cytoskeleton. Adherent cells pull on their substrate with actin-myosin bundles. As the stiffness of the substrate increases, so does the tension and concomitant bundling of the actin cytoskeleton. This tension is transferred into the nuclear lamina, where it alters nuclear shape and increases nucleocytoplasmic transport [Feldherr and Akin, 1993]. It is possible nucleocytoplasmic transport increases in a similar fashion under hypo-osmotic load as a result of tension introduced to the lamina by swelling of the nucleoplasm.
How do osmotic and mechanical stresses affect the genome?
It is now widely accepted that mechanical stresses also act on the genome through a biophysical pathway. Mechanical loads are transferred from extracellular matrix molecules via integrins to the actin cytoskeleton and intermediate filament network, which is in turn connected to the nuclear lamina. The nuclear lamina binds chromatin directly and via small proteins such as emerin [Dahl et al., 2008]. Therefore, there is a physical connection from the extracellular matrix to the genome along which mechanical stress can be transmitted [Maniotis et al., 1997]. Similarly, osmotic stress can also act on the genome via a direct, biophysical pathway.
How does extracellular osmolarity affect the nucleus?
One potential mechanism by which extracellular osmolarity may influence the nucleus is through alterations in intracellular macromolecular concentrations, which can influence nuclear size and chromatin condensation. Macromolecule concentration has a powerful influence on the nucleus due to a phenomenon known as the excluded volume effect. Macromolecules by definition have finite radius. This means that the center of the molecule is excluded not only from space occupied by another molecule but also from a region one radius deep surrounding that other molecule. This region is the excluded volume. Excluded volume effects can greatly accelerate reaction kinetics because they raise the effective concentration of a macromolecule by reducing the volume through which it diffuses (Fig. 2). For example, the effective concentration for hemoglobin under physiological levels of crowding (approximately 300 mg of solute per 1 ml of water) is 80 times the actual concentration [Minton, 2001]. As two molecules approach one another, their excluded volumes overlap, creating an attractive force that can be understood in entropic or osmotic terms. From an entropic perspective, overlap of excluded volumes reduces the total excluded volume in the system (Fig. 3A). This increases the volume available to other solute molecules, allowing them to occupy a greater number of position states and become more disordered. This gain in entropy outweighs the loss of entropy due to ordering of the aggregate. The attractive force between molecules in a crowded solution can equivalently be modeled as an osmotic pressure. There is an inaccessible region around the contact between two spherical molecules that can be thought of as an osmometer (Fig. 3B). The concentration is zero in this region because solute molecules are too large to enter it so osmotic pressure tends to draw water out of it to equilibrate it with the rest of the solution. This pressure creates an attractive force at the site of contact. This concept of osmotic pressure due to steric exclusion can be extended to the more general geometry of a porous gel permeated by a solution of macromolecules. The concentration of macromolecules is lower in the pore fluid of the gel than in the solution outside the gel as larger macromolecules are excluded from the smallest pores and channels in the gel. This difference in concentration is quantified by the partition coefficient, which is the ratio between the concentration in the solution and the concentration in the pore fluid of the gel. This difference leads to an osmotic gradient that draws water out of the gel. One important conclusion from this uneven partition model of osmotic pressurization is that the pressure on the gel increases with increasing concentration of the macromolecule solution [Albro et al., 2007].
What is the equilibrium osmolarity of the body?
The equilibrium osmolarity of the body is one of the most tightly controlled physiological parameters, regulated by a balance of hydration and solute concentrations [Bourque, 2008]. In addition to this system-level response, osmotic stress is a potent regulator of the normal function of cells that are exposed to osmotically active environments under physiologic or pathologic conditions. The ability at the cellular level to alter gene expression and metabolic activity in response to changes in the osmotic environment provides an additional regulatory mechanism for a diverse array of tissues and organs in the musculoskeletal system [Urban et al., 1993], kidney [Miyakawa et al., 1998], cardiovascular system [Zhou et al., 1997], and lung [Fedan et al., 1999], as well as in the protective response of cells subject to osmotic insult [Yancey et al., 1982], potentially following mechanical injury [Jayakumar et al., 2008].
WHAT ARE OSMOTIC LAXATIVES?
You may notice that the word “osmotic” is pretty close to “osmosis,” which means we’re talking about the movement of water. Osmotic laxatives, such as MiraLAX ®, attract water and keep it in your colon. This action allows MiraLAX ® to hydrate and soften stools, and unblock your system without causing harsh gastrointestinal side effects.
Do osmotic laxatives work?
The use of an osmotic laxative like MiraLAX ® can help relieve occasional constipation.
What is the process of osmosis?
Osmosis occurs when a solvent moves through a semi-permeable membrane to dilute a solute that is not able to permeate the membrane. The process is essential for the transport of water across biological membranes in living organisms.
How does water move in and out of a cell?
In order to maintain the cell's health, water moves in and out of the cell through the process of osmosis, diluting the cellular salt level. The process of osmosis can be observed during the rehydration of dried fruits and vegetables. While plant cells are not identical to animal cells, both cell types have a semi-permeable cell wall or membrane.
What is the most common solvent in osmosis?
In the process of osmosis, a less concentrated solvent moves through a semi-permeable membrane into a more concentrated solvent. The most common solvent is water. In animal cells, salt is not able to move in or out of the cellular membrane.
How is osmotic pressure determined?
Osmotic pressure is determined by solute concentration – water will “try harder” to diffuse into an area with a high concentration of a solute, such as a salt, than into an area with a low concentration. In reality of course, osmotic pressure is not a “desire” of water to move, but rather an extension of the natural law ...
Why do organisms use osmotic pressure?
Some organisms, such as plants that use osmotic pressure to move water, have taken advantage of this principle. But it can also threaten the health of cells and organisms when there is too much or too little water in the extracellular environment compared to the inside of the cell.
What is the meaning of osmosis pressure?
Osmotic Pressure Definition. Osmotic pressure can be thought of as the pressure that would be required to stop water from diffusing through a barrier by osmosis. In other words, it refers to how hard the water would “push” to get through the barrier in order to diffuse to the other side. Osmotic pressure is determined by solute concentration – ...
Why can't solutes move in osmosis?
So in the case of osmosis, the solutes cannot move because they cannot pass through the membrane. However, the water can move, and it does – passing through the membrane to an area with higher solute concentration. This can cause the total volume of water on each side of the membrane to change: the side of the membrane with more solutes may end up ...
What does hypertonic mean in science?
In scientific terms, they are “ hypertonic ” – which means “the concentration of solute is too high.”. Plants can also demonstrate the power of osmotic pressure as they grow. You may have seen plants springing up through asphalt, or tree roots growing through bricks or concrete.
Does osmotic pressure cause water to move?
In reality of course, osmotic pressure is not a “desire” of water to move, but rather an extension of the natural law that all matter will become randomly distributed over time. When the concentrations of substances are different in two areas and the areas have contact with each other, the random motion of particles will cause the substances to diffuse until the solution is uniform throughout the whole area.
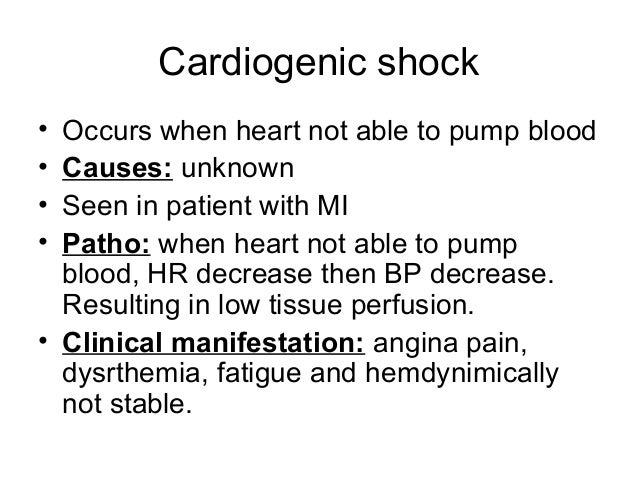