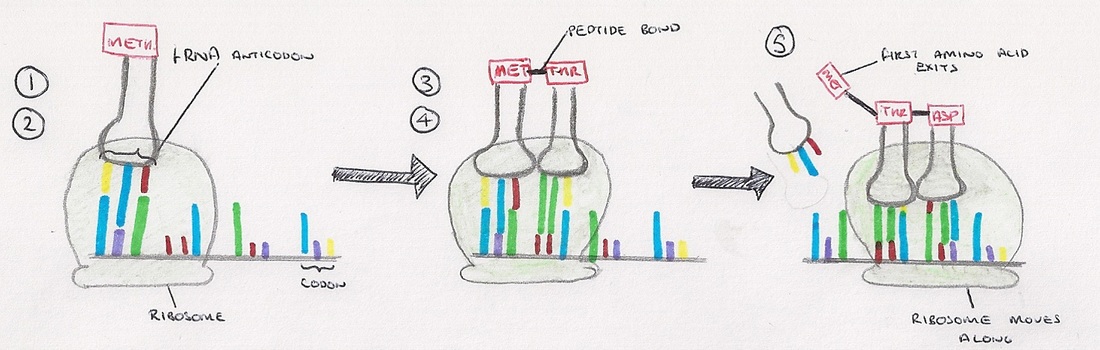
What do motor proteins do in a cell?
Many motor proteins carry membrane-enclosed organelles—such as mitochondria, Golgi stacks, or secretory vesicles—to their appropriate locations in the cell. Other motor proteins cause cytoskeletal filaments to slide against each other, generating the force that drives such phenomena as muscle contraction, ciliary beating, and cell division.
How do motor proteins make unidirectional motion?
For such unidirectional motion, a motor proteinmust use the energy derived from ATP binding and hydrolysis to force a large movement in part of the protein molecule.
How do motor proteins transport cargo?
Similar to a freight train, motor proteins capable of carrying cargo also face restrictions in the direction they can travel, the speed at which they travel and the possibility of traffic jams along the route. Specific members of the Myosin superfamily of motor proteins are known to transport cargo along actin filaments.
What is the function of myosin motor proteins?
MYOSIN Myosins form a superfamily of molecular motor proteins that power muscle contraction, as well as movement on actin filaments in all eukaryotic cells. All known myosin superfamily members follow the blueprint shown in Figure 1(Odronitz and Kollmar 2007).
What do motor proteins interact with?
Motor proteins are the driving force behind muscle contraction and are responsible for the active transport of most proteins and vesicles in the cytoplasm. They are a class of molecular motors that are able to move along the surface of a suitable substrate, powered by the hydrolysis of ATP.
Do motor proteins bind to actin?
All myosin motors hydrolyze ATP, but, when not bound to actin, only slowly release the hydrolysis products, MgADP and inorganic phosphate (Pi). Binding to an actin filament stimulates myosin to release Pi, followed by the release of Mg and ADP.
Do motor proteins bind to microtubules?
The heavy chains have long α-helical regions that wind around each other in a coiled-coil structure. The amino-terminal globular head domains of the heavy chains are the motor domains of the molecule: They bind to both microtubules and ATP, the hydrolysis of which provides the energy required for movement.
How do motor proteins bind to vesicles?
These proteins bind to vesicles and organelles and use energy from ATP to move them along the microtubule or microfilament network. Two families of motor proteins, the kinesins and dyneins, move vesicles along microtubules, and members of the myosin family move them along microfilaments (see Box 1).
Are actin and myosin motor proteins?
Actin motors Myosins are a superfamily of actin motor proteins that convert chemical energy in the form of ATP to mechanical energy, thus generating force and movement. The first identified myosin, myosin II, is responsible for generating muscle contraction.
What are motor proteins quizlet?
motor proteins. category of cellular proteins that use ATP as a source of energy to promote movement; (Microtubules) 1.
How can motor protein walk on microtubules?
Kinesins move across microtubules by hydrolyzing one molecule of ATP at each step in a single direction to produce a “walking” motion.
How do motor proteins transport cargo along cytoskeleton?
Kinesin transports cargo along microtubules The kinesin protein contains a head domain that binds to microtubules, a stalk region where two kinesin monomers interact and dimerize, and a cargo-binding domain that allows the protein to facilitate cytoskeleton-dependent intracellular transport.
How do motor proteins move on microtubule tracks?
Myosin motors move on actin filaments, whereas kinesin and dynein motors move on microtubules. These molecular motor proteins all convert the energy from ATP into force and movement on either the actin or microtubule tracks. Myosin and kinesin had a common ancestor related to GTPases, but dynein is an AAA ATPase.
What do motor proteins do in neurons?
Inside neurons, motor proteins haul precious cargo, moving essential goods along thread-like roadways called microtubule tracks. Inside neurons, motor proteins haul precious cargo, moving essential goods along thread-like roadways called microtubule tracks.
How does a motor protein walk?
Motor proteins fulfill the role of transporting large cargo about the cell to their required destinations. Kinesins are motor proteins that transport such cargo by walking unidirectionally along microtubule tracks hydrolysing one molecule of adenosine triphosphate (ATP) at each step.
How do motor proteins use ATP?
Perhaps the most fascinating proteins that associate with the cytoskeleton are the molecular motors called motor proteins. These remarkable proteins bind to a polarized cytoskeletal filament and use the energy derived from repeated cycles of ATP hydrolysis to move steadily along it.
Why are motor proteins important?
The importance of motor proteins in cells becomes evident when they fail to fulfill their function. For example, kinesin deficiencies have been identified as the cause for Charcot-Marie-Tooth disease and some kidney diseases. Dynein deficiencies can lead to chronic infections of the respiratory tract as cilia fail to function without dynein. Numerous myosin deficiencies are related to disease states and genetic syndromes. Because myosin II is essential for muscle contraction, defects in muscular myosin predictably cause myopathies. Myosin is necessary in the process of hearing because of its role in the growth of stereocilia so defects in myosin protein structure can lead to Usher syndrome and non-syndromic deafness.
What is a motor protein?
Jump to navigation Jump to search. Kinesin walking on a microtubule using protein dynamics on nanoscales. Motor proteins are a class of molecular motors that can move along the cytoplasm of animal cells. They convert chemical energy into mechanical work by the hydrolysis of ATP.
What is a dynein motor?
Dyneins are microtubule motors capable of a retrograde sliding movement. Dynein complexes are much larger and more complex than kinesin and myosin motors. Dyneins are composed of two or three heavy chains and a large and variable number of associated light chains. Dyneins drive intracellular transport toward the minus end of microtubules which lies in the microtubule organizing center near the nucleus. The dynein family has two major branches. Axonemal dyneins facilitate the beating of cilia and flagella by rapid and efficient sliding movements of microtubules. Another branch is cytoplasmic dyneins which facilitate the transport of intracellular cargos. Compared to 15 types of axonemal dynein, only two cytoplasmic forms are known.
Which proteins move along the cytoskeleton?
Motor proteins utilizing the cytoskeleton for movement fall into two categories based on their substrate: microfilaments or microtubules. Actin motors such as myosin move along microfilaments through interaction with actin, and microtubule motors such as dynein and kinesin move along microtubules through interaction with tubulin.
Why is myosin II important?
Because myosin II is essential for muscle contraction, defects in muscular myosin predictably cause myopathies. Myosin is necessary in the process of hearing because of its role in the growth of stereocilia so defects in myosin protein structure can lead to Usher syndrome and non-syndromic deafness.
What is the function of myosins?
The first identified myosin, myosin II, is responsible for generating muscle contraction. Myosin II is an elongated protein that is formed from two heavy chains with motor heads ...
What are the two types of microtubule motors?
There are two basic types of microtubule motors: plus-end motors and minus-end motors, depending on the direction in which they "walk" along the microtubule cables within the cell.
What is the function of motor proteins?
Motor proteins drive active ATP-dependent motion of organelles along cytoskeletal filaments, a process that is necessary for protein homeostasis, secretion, and for many other vital cellular functions (Barlan, Rossow, & Gelfand, 2013; Vale, 2003 ).
What proteins are involved in motors?
A number of proteins, such as dynactin, Lis1, kinesin accessory proteins, miro–milton, JIP, etc., interact with MT motors to regulate their motion ( Verhey & Hammond, 2009; Vallee, McKenney, & Ori-McKenney, 2012 ). This regulation controls many motor properties such as ATPase rate, affinity for cargo or filament, force generation, geometrical arrangement of motors on the cargo, as well as other properties. The sum total of this regulation is manifested as the motion of organelles inside cells, which can be easily observed. However, the interpretation of this motion is often difficult because: (1) The microarchitecture of the dense cytoskeletal network is unknown and likely modulates the motion in unpredictable manner, (2) Additional protein factors besides motors and their binding partners may influence motion (e.g., MT binding proteins). Furthermore, interfering with the function of specific motors/motor-associated proteins in vivo by RNAi or dominant negative constructs may lead to unknown pleiotropic effects.
How are biotinylated dynein motor proteins attached to a gold surface?
Biotinylated dynein motor proteins were specifically attached to a gold surface via biotin–streptavidin linkage and thorough blocking of the other surfaces. The glass surfaces were blocked with 0.2 mg/ml PLL-PEG (SurfaceSolutions, Switserland) for 5 min, rinsed with MRB80, blocked with 1 mg/ml κ-casein in MRB80 for 5 min, and rinsed. Subsequently, a multilayer (to enhance the number of binding sites) of biotinylated-BSA and streptavidin was formed ( Romet-Lemonne et al., 2005) on the gold barrier ( Fig. 6 B). A streptavidin mix (0.5 mg/ml streptavidin, 1 mg/ml κ-casein, 5 mg/ml BSA in MRB80) and a biotinylated-BSA-mix (1.5 mg/ml biotinylated-BSA, 1 mg/ml κ-casein, 5 mg/ml BSA in MRB80) were sequentially introduced and incubated for 5 min to create a multilayer with 3 layers of streptavidin and 2 of biotinylated-BSA. Afterward the dynein was introduced (20 nM dynein, 1 mg/ml κ-casein, 5 mg/ml BSA in MRB80) for 5 min (in the case of control experiments without dynein, this step was left out).
What is the role of helicases in DNA replication?
Helicases are motor proteins that move unidirectionally on nucleic acids by deriving energy from concerted and coordinated NTP hydrolysis reaction. A class of helicases, ring shaped hexameric helicases, plays essential roles in DNA replication, repair, recombination, and transcription.
What is the function of dyneins?
Dyneins were first identified by Gibbons as the adenosine triphosphatases (ATPases) which are responsible for the propulsive flagella bending and he named them for the unit of force, the dyne. Dynein family members have been found in eukaryotic organisms from yeast to humans. They are members of the AAA + ATPase superfamily. All dyneins are large protein complexes composed of one or more approximately 530,000 Dalton (Da) polypeptides, known as heavy chains, and a variety of accessory subunits from 10,000 to 150,000 Da.
How does a cytoskeletal motor approach stall?
There are three general ways that a cytoskeletal motor can approach stall: (1) it can detach before reaching stall, (2) it can stop stepping altogether as it approaches the stall force (static stall), or (3) it can step forward and backward around the stall force (dynamic stall). To transport cargo, any of the three options are acceptable since the motor has an opportunity to reengage a track after a failed run. However, to regulate the tension of cytoskeletal filaments, the best option is (3), dynamic stall. As the motor experiences superstall loads, it can relax to the original stall force without detaching and suddenly dissipating tension. In contrast, a static stall motor acts more like a ratchet, reeling in slack filaments but stubbornly holding on as load increases. Likewise, detaching before the stall force is reached is a poor option for a cytoskeletal motor that regulates tension, since the detachment force will vary considerably with each run.
What is myosin in eukaryotic cells?
Myosins are motor proteins that are present in virtually all eukaryotic cells; indeed the recent explosion in genomic information shows that there are at least 24 different families of myosin in higher organisms. The first myosin to be isolated, now called myosin II, is the protein in muscles that, together with actin, brings about contraction. It is a molecular machine that converts the chemical free energy of adenosine triphosphate (ATP) to mechanical force and movement. Studies, by both “traditional” and “single molecule” approaches, have been focused on understanding the mechanism of this energy conversion and the diversity of functions of myosins in cells.
How do cytoskeletal motor proteins work?
Thus, cytoskeletal motor proteins work in a manner highly analogous to GTP-binding proteins, except that in motor proteins the small proteinconformational changes (a few tenths of a nanometer) associated with nucleotidehydrolysis are amplified by special protein domains—the lever arm in the case of myosin and the linker in the case of kinesin—to generate large-scale (several nanometers) conformational changes that move the motor proteins stepwise along their filament tracks. The analogy between the GTPases and the cytoskeletal motor proteins has recently been extended by the observation that one of the GTP-binding proteins—the bacterial elongation factorG—translates the chemical energy of GTP hydrolysis into directional movement of the mRNA moleculeon the ribosome.
What is the first motor protein?
The first motor proteinidentified was skeletal muscle myosin, which is responsible for generating the force for muscle contraction. This myosin, called myosin II(see below) is an elongated protein that is formed from two heavy chains and two copies of each of two light chains. Each of the heavy chains has a globular head domainat its N-terminus that contains the force-generating machinery, followed by a very long amino acidsequence that forms an extended coiled-coilthat mediates heavy chain dimerization (Figure 16-51). The two light chains bind close to the N-terminal head domain, while the long coiled-coil tail bundles itself with the tails of other myosin molecules. These tail-tail interactions result in the formation of large bipolar “thick filaments” that have several hundred myosin heads, oriented in opposite directions at the two ends of the thick filament (Figure 16-52).
How does myosin move?
Most strikingly, a single dimer of conventional kinesin moves in a highly processive fashion, traveling for hundreds of ATPasecycles along a microtubulewithout dissociating. Skeletal muscle myosin II, in contrast, cannot move processively and makes just one or a few steps along an actinfilament before letting go. These differences are critical for the motors' various biological roles. A small number of kinesin molecules must be able to transport a mitochondrion all the way down a nerve cellaxon, and therefore require a high level of processivity. Skeletal muscle myosin, in contrast, never operates as a single moleculebut rather as part of a huge array of myosin II molecules. Here processivity would actually inhibit biological function, since efficient muscle contraction requires that each myosin head perform its power stroke and then quickly get out of the way, to avoid interfering with the actions of the other heads attached to the same actin filament.
What is the fastest molecular motor?
Dyneins are the largest of the known molecular motors, and they are also among the fastest: axonemal dyneins can move microtubules in a test tube at the remarkable rate of 14 μm/sec. In comparison, the fastest kinesins can move their microtubules at about 2–3 μm/sec.
What are dyneins in a cell?
The dyneinfamily has two major branches (Figure 16-56). The most ancient branch contains the cytoplasmic dyneins ,which are typically heavy-chain homodimers, with two large motor domains as heads. Cytoplasmic dyneins are probably found in all eucaryotic cells, and they are important for vesicletrafficking, as well as for localization of the Golgi apparatus near the center of the cell. Axonemal dyneins,the other large branch, include heterodimers and heterotrimers, with two or three motor-domain heads, respectively. They are highly specialized for the rapid and efficient sliding movements of microtubules that drive the beating of cilia and flagella (discussed later). A third, minor, branch shares greater sequence similarity with cytoplasmic than with axonemal dyneins but seems to be involved in the beating of cilia.
What are the differences between myosin and kinesin?
The motor domainof myosins is substantially larger than that of ki nesins, about 850 amino acids compared with about 350. The two classes of motor proteins track along different filaments and have different kinetic properties, and they have no identifiable amino acidsequence similarities. However, determination of the three-dimensional structure of the motor domains of myosin and kinesinhas revealed that these two motor domains are built around nearly identical cores (Figure 16-57). The central force-generating element that the two types of motor proteins have in common includes the site of ATP binding and the machinery necessary to translate ATP hydrolysis into an allosteric conformational change. The differences in domain size and in the choice of track can be attributed to large loops extending outward from this central core. These loops include the actin-binding and microtubule-binding sites, respectively.
What are the proteins that move the cytoskeleton?
Perhaps the most fascinating proteins that associate with the cytoskeleton are the molecular motors called motor proteins . These remarkable proteins bind to a polarized cytoskeletal filament and use the energy derived from repeated cycles of ATP hydrolysis to move steadily along it. Dozens of different motor proteins coexist in every eucaryotic cell. They differ in the type of filament they bind to (either actin or microtubules), the direction in which they move along the filament, and the “cargo” they carry. Many motor proteins carry membrane-enclosed organelles—such as mitochondria, Golgi stacks, or secretory vesicles—to their appropriate locations in the cell. Other motor proteins cause cytoskeletal filaments to slide against each other, generating the force that drives such phenomena as muscle contraction, ciliary beating, and cell division.
What is the function of motor proteins?
Image credit: OpenStax Biology. Motor proteins use energy in the form of ATP to “walk” along specific cytoskeletal tracks. They are essential for movement of vesicles and other cargoes within cells, as well as for the movement of muscle and cilia/flagella: Myosin is associated with actin microfilaments and is required for movement of muscle.
What are the proteins that move the cilia?
ATP, dynein motor proteins, and microtubule tracks are essential for movement of eukaryotic cilia and flagella. Flagella (singular, flagellum) are long, hair-like structures that extend from the cell surface and are used to move an entire cell, such as a sperm. If a cell has any flagella, it usually has one or just a few.
Why does myosin stay stuck after a power stroke?
Because ATP is required for myosin to be able to release the actin, depletion of ATP due to muscle fatigue will cause muscles to remain locked in a contracted state; this is thought to be one of several sources of cramping after exercise.
How does muscle shortening work?
How does this process work? The motion of muscle shortening occurs as myosin heads bind to actin and pull the actin inwards. This action requires energy, which is provided by ATP. Myosin binds to actin at a binding site on the globular actin protein. Myosin has another binding site for ATP at which enzymatic activity hydrolyzes ATP to ADP, releasing an inorganic phosphate molecule and energy. The process works in a cycle (called the “cross bridge cycle”) like this:
What is the function of microfilaments?
Microfilaments (also called actin filaments) have a double helix-like structure composed of actin protein subunits; microfilaments serve as tracks for the motor protein myosin and are involved in many cellular processes that require motion.
What is a sarcomere?
A sarcomere is the region from one Z line to the next Z line. Many sarcomeres are present in a myofibril, resulting in the striation pattern characteristic of skeletal muscle. Image credit: OpenStax Biology. The protein components of the sarcomere include actin, myosin, tropomyosin, and troponin:
What are the components of a multicellular animal?
In multicellular animals, these components are the skeleton and muscles. In single-celled animals and individual cells, these components are often flagella and/or cilia. All of these structures rely on both motor proteins and components of the cytoskeleton. The cytoskeleton (literally, “cell skeleton”) is a network of filaments ...
Talk Overview
Molecular motor proteins are fascinating enzymes that power much of the movement performed by living organisms. In this introductory lecture, I will provide an overview of the motors that move along cytoskeletal tracks (kinesin and dynein which move along microtubules and myosin which moves along actin).
Speaker Bio
Professor of the Department of Cellular and Molecular Pharmacology; Investigator in the Howard Hughes Medical Institute University of California, San Francisco Continue Reading
Which family of proteins transports cargo along actin filaments?
While the myosin family of proteins transport cargo along actin filaments, a different group of proteins utilize microtubules to faciliate a similar process. Here, we consider the kinesin superfamily.
Which protein transports cargo along microtubules?
Kinesin transports cargo along microtubules. While the myosin family of proteins transport cargo along actin filaments, a different group of proteins utilize microtubules to faciliate a similar process. Here, we consider the kinesin superfamily. The kinesin protein contains a head domain that binds to microtubules, ...
How does the neck linker work in a powerstroke?
In the initial stage of the powerstroke cycle, the neck linker of the leading head is tethered to the head but extended backwards and not interacting with the docking site (A) Following ATP binding and the subsequent conformational change, the neck linker docks with its docking site in the leading head. This pulls the trailing head forward (B). The trailing head continues forward until the neck-linker of the trailing head disengages the docking site. This head finds the next binding site and becomes the leading head (C). The cycle continues, allowing the Kinesin to “walk” down the microtubule filament.
What is the function of myosin X?
Myosin-X facilitates the transport of components from the cell body to filopodial tips, resulting in their integration into the cell membrane as receptors/adhesions or their use in the growth of actin filaments and filopodia [1] [2] [3] [4] [5].
How does myosin X move?
Movement of myosin-X is driven by ATP hydrolysis, in a unique mechanism that resembles walking or stepping. This movement is known to occur preferentially on actin bundles rather than single actin filaments [6] [7]. Although it is essentially a forward movement, evidence indicates that the protein may also take side-steps. This may be carried out as a means of overcoming obstacles or defects in the track [7].
How do kinesins move?
Conventional kinesins move along microtubule filaments in a manner that resembles human walking. This has been described as an asymmetric ‘hand-over-hand’ mechanism where one head domain steps forward ~16.2nm whilst the other head remains stationary.
Which is longer, microtubules or kinesins?
Microtubules are generally much longer than actin filaments and as such kinesin can transport cargo over significantly longer distances than the myosins [9]. In their inactive state the kinesins exist as monomers, each possessing an N-terminal head (motor region), a neck linker and a globular tail domain.

Overview
Cytoskeletal motor proteins
Motor proteins utilizing the cytoskeleton for movement fall into two categories based on their substrate: microfilaments or microtubules. Actin motors such as myosin move along microfilaments through interaction with actin, and microtubule motors such as dynein and kinesin move along microtubules through interaction with tubulin.
There are two basic types of microtubule motors: plus-end motors and minus-end motors, depen…
Cellular functions
The best prominent example of a motor protein is the muscle protein myosin which "motors" the contraction of muscle fibers in animals. Motor proteins are the driving force behind most active transport of proteins and vesicles in the cytoplasm. Kinesins and cytoplasmic dyneins play essential roles in intracellular transport such as axonal transport and in the formation of the spindle apparatus and the separation of the chromosomes during mitosis and meiosis. Axonemal dynein, …
Diseases associated with motor protein defects
The importance of motor proteins in cells becomes evident when they fail to fulfill their function. For example, kinesin deficiencies have been identified as the cause for Charcot-Marie-Tooth disease and some kidney diseases. Dynein deficiencies can lead to chronic infections of the respiratory tract as cilia fail to function without dynein. Numerous myosin deficiencies are related to disease states and genetic syndromes. Because myosin II is essential for muscle contraction, …
Other molecular motors
Besides the motor proteins above, there are many more types of proteins capable of generating forces and torque in the cell. Many of these molecular motors are ubiquitous in both prokaryotic and eukaryotic cells, although some, such as those involved with cytoskeletal elements or chromatin, are unique to eukaryotes. The motor protein prestin, expressed in mammalian cochlear outer hair cells, produces mechanical amplification in the cochlea. It is a direct voltage-to-force converter, …
See also
• ATP synthase
• Cytoskeleton
• Protein dynamics
External links
• MBInfo - What are Motor Proteins?
• Ron Vale's Seminar: "Molecular Motor Proteins"
• Biology of Motor Proteins Institute for Biophysical Chemistry, Göttingen
• Jonathan Howard (2001), Mechanics of motor proteins and the cytoskeleton. ISBN 9780878933334