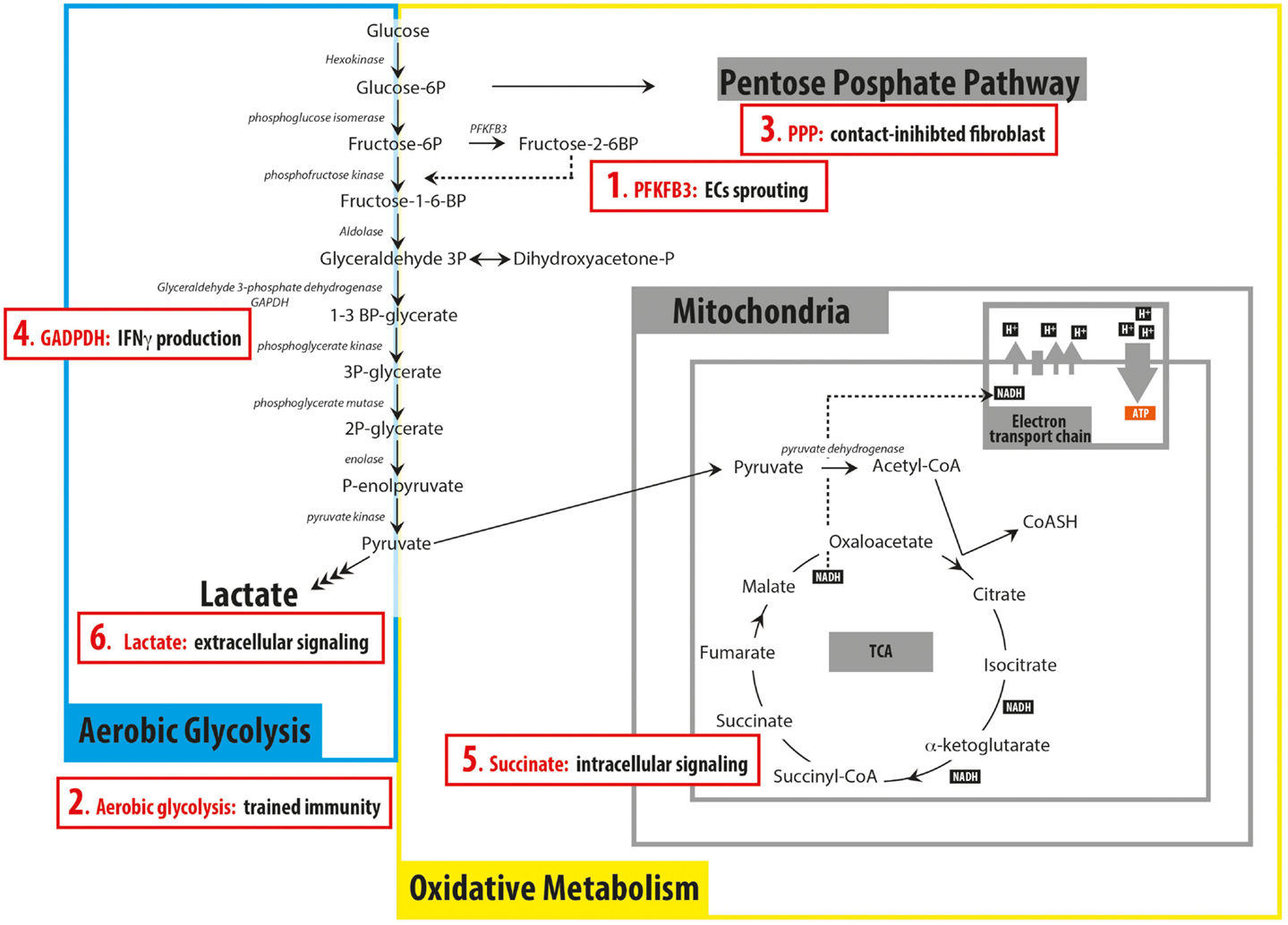
What is regulation of metabolic pathways?
Regulation of Metabolic Pathways: Which Are Optimal? Exquisite mechanisms have evolved that control the flux of metabolites through metabolic pathways to insure that the output of the pathways meets biological demand and that energy in the form of ATP is not wasted by having opposing pathways run concomitantly in the same cell.
How do exquisite metabolic pathways work?
Exquisite mechanisms have evolved that control the flux of metabolites through metabolic pathways to insure that the output of the pathways meets biological demand and that energy in the form of ATP is not wasted by having opposing pathways run concomitantly in the same cell.
How do you regulate the activity of an enzyme?
A. Changing the activity of a pre-existing enzyme: The quickest way to modulate the activity of an enzyme is to alter the activity of an enzyme that already exists in the cell. The list below, illustrated in the following figure, gives common ways to regulate enzyme activity
How can feedback inhibition be energetically advantageous to a cell?
Likewise it be energetically advantageous to a cell if the end product of an entire pathway could likewise bind to the initial enzyme in the pathways and inhibit it, allowing the whole pathway to be inhibited. This type of feedback inhibition is commonly oberved
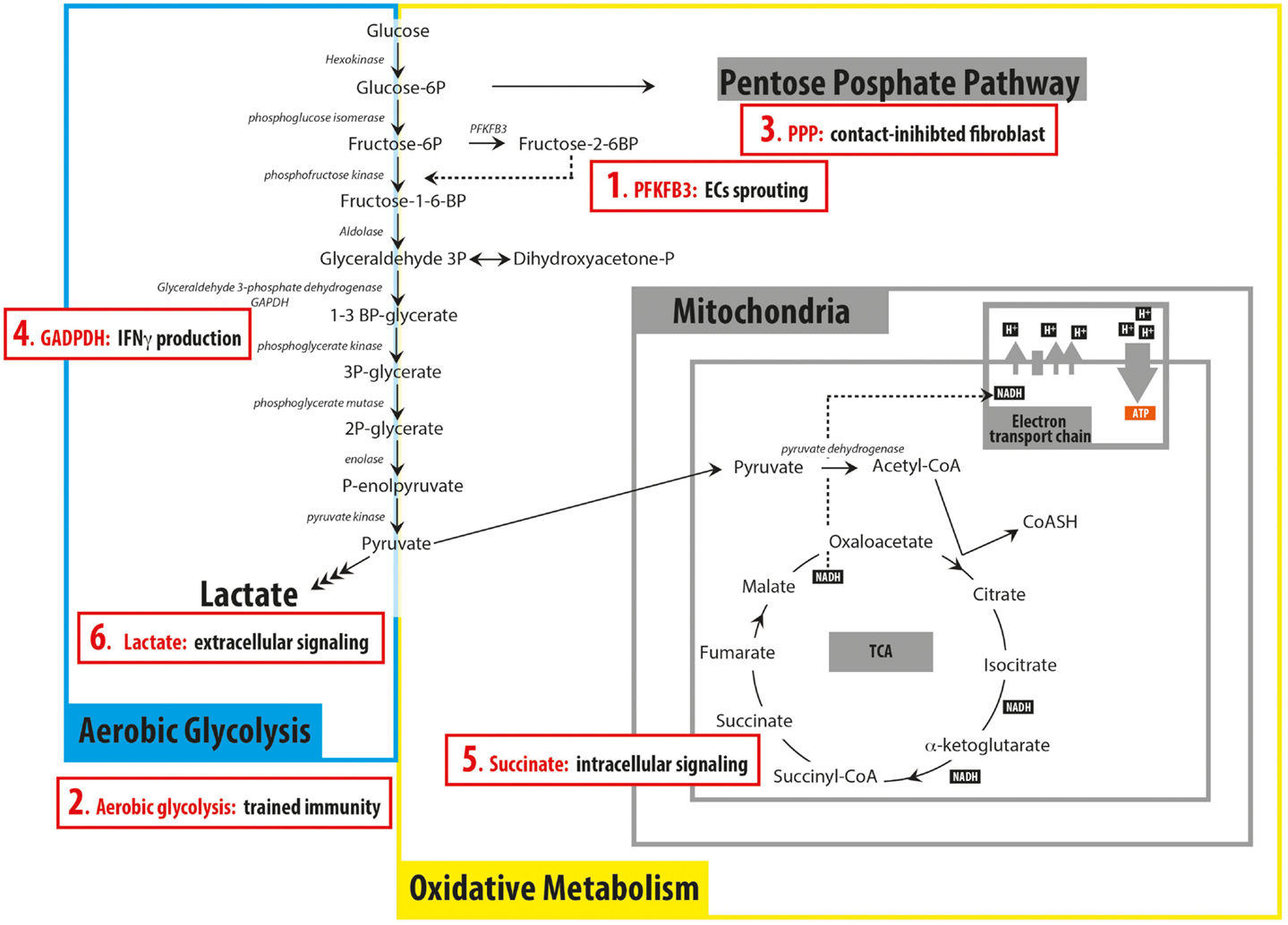
How do cells regulate metabolic flux?
Organisms and cells have evolved systems to modulate metabolic flux over short and long time scales. Though not discussed in detail here, hormones and other extracellular factors communicate signals between tissues to regulate metabolic function ( Randle, 1963; Saltiel and Kahn, 2001 ). At the cellular level, genes encoding enzyme isoforms and regulatory factors allow tissue- and context-specific responses, and the abundance of these proteins is controlled by messenger RNA (mRNA) transcription, splicing, stability, and translation ( Cairns et al., 2011 ). Another means of regulating enzyme function is posttranslational modifications (PTMs), which provide a feedback mechanism for metabolites that act as substrates for PTM reactions ( Zhao et al., 2010 ). Finally, small molecules can affect metabolic flux by allosteric effects on enzymes ( Carminatti et al., 1971 ). The goal of these processes is to parse metabolites through pathways in the proportions needed to match the requirements of individual cells.
How does metabolism affect cellular function?
Metabolism impacts all cellular functions and plays a fundamental role in biology. In the last century, our knowledge of metabolic pathway architecture and the genomic landscape of disease has increased exponentially. Combined with these insights, advances in analytical methods for quantifying metabolites and systems approaches to analyze these data now provide powerful tools to study metabolic regulation. Here we review the diverse mechanisms cells use to adapt metabolism to specific physiological states and discuss how metabolic flux analyses can be applied to identify important regulatory nodes to understand normal and pathological cell physiology.
What is the signaling response to low oxygen?
A signaling response to low oxygen is mediated by the EGLN-family of alpha-ketoglutarate (αKG)-dependent dioxygenases. The half-life of the hypoxia-inducible factor (HIF) transcription factor complex α subunit is short when oxygen levels are high because the protein is hydroxylated by the EGLN proteins, recognized by the von Hippel Lindau E3 ubiquitin ligase, and targeted for degradation ( Ivan et al., 2001; Jaakkola et al., 2001 ). In hypoxia, oxygen becomes limiting for protein hydroxylation, and active HIF transcription factor accumulates to induce an adaptive gene expression program ( Kaelin and Ratcliffe, 2008 ). In addition, because the enzymatic mechanism of HIFα modification by EGLN proteins requires O 2, αKG, and Fe 2+ while producing both succinate and the hydroxylated species ( Figure 3 ), O 2 is not the only factor that influences enzyme activity and HIFα stabilization ( Bruick and McKnight, 2001; Epstein et al., 2001 ). Changes in cellular redox state can increase HIFα levels because increased ROS oxidizes Fe 2+ and limits EGLN activity, and elevated ROS levels during hypoxia can activate HIF-dependent transcription ( Chandel et al., 1998 ). Emerging evidence suggests that other αKG-dependent dioxygenases in the TET and JmjC protein families can hydroxylate DNA and histones to influence the epigenetic state of cells ( Tahiliani et al., 2009; Tsukada et al., 2006) ( Figure 3 ). In turn, αKG-dependent dioxygenase activity can be directly modulated by metabolite levels. Succinate and fumarate abundances increase with oncogenic loss-of-function mutations in the TCA cycle enzymes SDH and FH, and both metabolites can affect dioxygenase activity ( Xiao et al., 2012 ). For the same reason, changes in nutrient levels or regulatory events that lead to alterations in αKG/succinate ratios might also influence HIF-dependent transcription ( Tennant et al., 2009 ). ( D )-2-hydroxyglutarate (2HG), an oncometabolite that accumulates to high levels in cells as a direct product of cancer-associated mutant IDH enzymes, can inhibit αKG-dependent dioxygenase activity ( Chowdhury et al., 2011; Figueroa et al., 2010; Xu et al., 2011 ). This effect of 2HG can block differentiation of cells by inhibition of TET2 and other αKG-dependent dioxygenases that regulate chromatin structure and collagen maturation and has been implicated in the pathogenesis of IDH mutant tumors ( Figueroa et al., 2010; Lu et al., 2012; Sasaki et al., 2012a; Sasaki et al., 2012b ). Increasing the complexity of this metabolic regulation, 2HG can also replace αKG as cosubstrate for a subset of these dioxygenases ( Koivunen et al., 2012 ). These findings illustrate how multiple aspects of metabolism can influence αKG-dependent dioxygenase enzyme activity and have effects on cell physiology, including promotion of cancer.
What is the function of oxidative PPP?
The oxidative PPP helps supply cells with NADPH to maintain reduced glutathione and mitigate damage caused by reactive oxygen species (ROS). Glucose-6-phosphate dehydrogenase (G6PD) catalyzes the first committed step in the oxidative PPP and directly converts NADP + to NADPH. Because these cofactors are a product and substrate of the enzyme, a falling NADPH/NADP + ratio stimulates flux through G6PD ( Holten et al., 1976 ). However, additional regulatory events can also influence flux through this pathway that branches from glycolysis just above the reaction catalyzed by phosphofructokinase (PFK1) ( Figure 2 ). PFK1 activity is responsive to levels of the metabolite fructose-2,6-bisphosphate (F2,6BP), and F2,6BP abundance is controlled by 6-phosphofructo-2-kinase/fructose-2,6-bisphosphate-2-phosphatase (PFK2), a bifunctional enzyme system designed to regulate F2,6BP levels and PFK1 in response to signaling inputs ( Pilkis et al., 1995 ). While this system forms a key circuit in the liver to control glycolysis and gluconeogenesis, selective expression of PFK2 isoforms with differential kinase and phosphatase activity can also impact PFK1 activity in other cells. In fact, expression of the PFKFB4 isoform of PFK2 is critical for redox homeostasis and cell survival in some prostate cancers ( Ros et al., 2012 ). Another enzyme that influences F2,6BP abundance is the fructose-2,6-bisphosphate-2-phosphatase TIGAR, which is a target of p53 in response to stress. Expression of TIGAR leads to decreased F2,6BP levels and PFK1 activity, which shunts more glucose carbon into the oxidative PPP ( Bensaad et al., 2006 ). Additionally, p53 can interact with G6PD and modulate oxidative PPP activity ( Jiang et al., 2011 ). Glycosylation of PFK1 itself also affects enzyme activity and regulates glucose flux to the oxidative PPP, potentially allowing a more rapid stress response than new enzyme synthesis ( Yi et al., 2012 ). More distal regulatory events in glycolysis can also influence PPP flux, as ROS-mediated oxidation of cysteine residues in the pyruvate kinase M2 isoform (PKM2) can acutely divert glycolytic flux toward the PPP ( Anastasiou et al., 2011 ). Finally, antioxidant response pathways such as NRF2 are triggered to activate transcriptional programs that increase expression of PPP and other enzymes that scavenge ROS ( Mitsuishi et al., 2012 ). It is likely that different combinations of the above strategies facilitate oxidative stress responses for cells in different situations.
What is the function of AMPK?
At the signal transduction level, AMP-activated protein kinase (AMPK) is a sensor that responds to changes in the ratio of ATP to AMP (and ADP) and coordinates diverse metabolic responses ( Hardie, 2011; Oakhill et al., 2011 ). AMPK is a heterotrimeric complex with each subunit encoded by more than one gene, and tissue-specific expression of different isoforms provides a genetically encoded means of mediating heterogeneous regulatory responses ( Viollet et al., 2009 ). AMPK affords both short- and long-term feedback control for cells by controlling the activity of numerous proteins through phosphorylation, including glucose transporters, 3-hydroxy-3-methyl-glutaryl-CoA reductase, and AcCoA carboxylase ( Hardie, 2011 ). By increasing glucose uptake and decreasing cholesterol and lipid synthesis, this regulation provides a means of rapidly modulating ATP production and consumption, respectively. AMPK also regulates the mammalian target of rapamycin complex-1 (mTORC1) ( Inoki et al., 2003 ). Inhibition of mTORC1 is crucial for cell survival under conditions of stress, as rapamycin-mediated inhibition can decrease biosynthetic processes that consume ATP and prevent bioenergetic catastrophe ( Choo et al., 2010 ). Additionally, activation of autophagy through phosphorylation of ULK1 can provide additional fuel to support ATP production in mitochondria ( Egan et al., 2011 ). Metabolic control over longer time scales is accomplished by AMPK through control of gene expression. SREBP1 is involved in lipid and carbohydrate metabolism, and AMPK is thought to inactivate this transcription factor through phosphorylation ( Li et al., 2011 ). Another important target of AMPK is PGC1α, which acts as a transcriptional coactivator of PPARγ to drive mitochondrial biogenesis ( Jäger et al., 2007 ). In this manner, AMPK induces changes in the cell that facilitate ATP generation. Together, these short- and long-term controllers mediate a coordinated response to stabilize the energy state of cells.
Can pyruvate be converted to lactate?
Increased LDH activity and/or decreased PDH activity can shunt glucose-derived pyruvate to lactate. However, metabolism of glucose to pyruvate requires conversion of NAD + to NADH, and NAD + must be regenerated from NADH for glycolysis to continue. Pyruvate to lactate conversion efficiently recycles NADH back to NAD +, while oxidation of NADH to NAD + involving the mitochondria requires four separate enzymatic reactions (numbered 1–4), metabolite transport across the mitochondrial membranes (A) and coupling to the electron transport chain (B) (or other routes of mitochondrial NADH oxidation).
What are the metabolic networks of cells?
Cells express lineage-specific networks of metabolic enzymes and regulatory factors that support appropriate tissue functions. Microenvironments, hormonal cues, and/or pharmacological perturbations will elicit adaptive metabolic responses that are unique to the metabolic networks of individual cells. Thus, a diverse set of metabolic phenotypes is observed in normal and disease states.
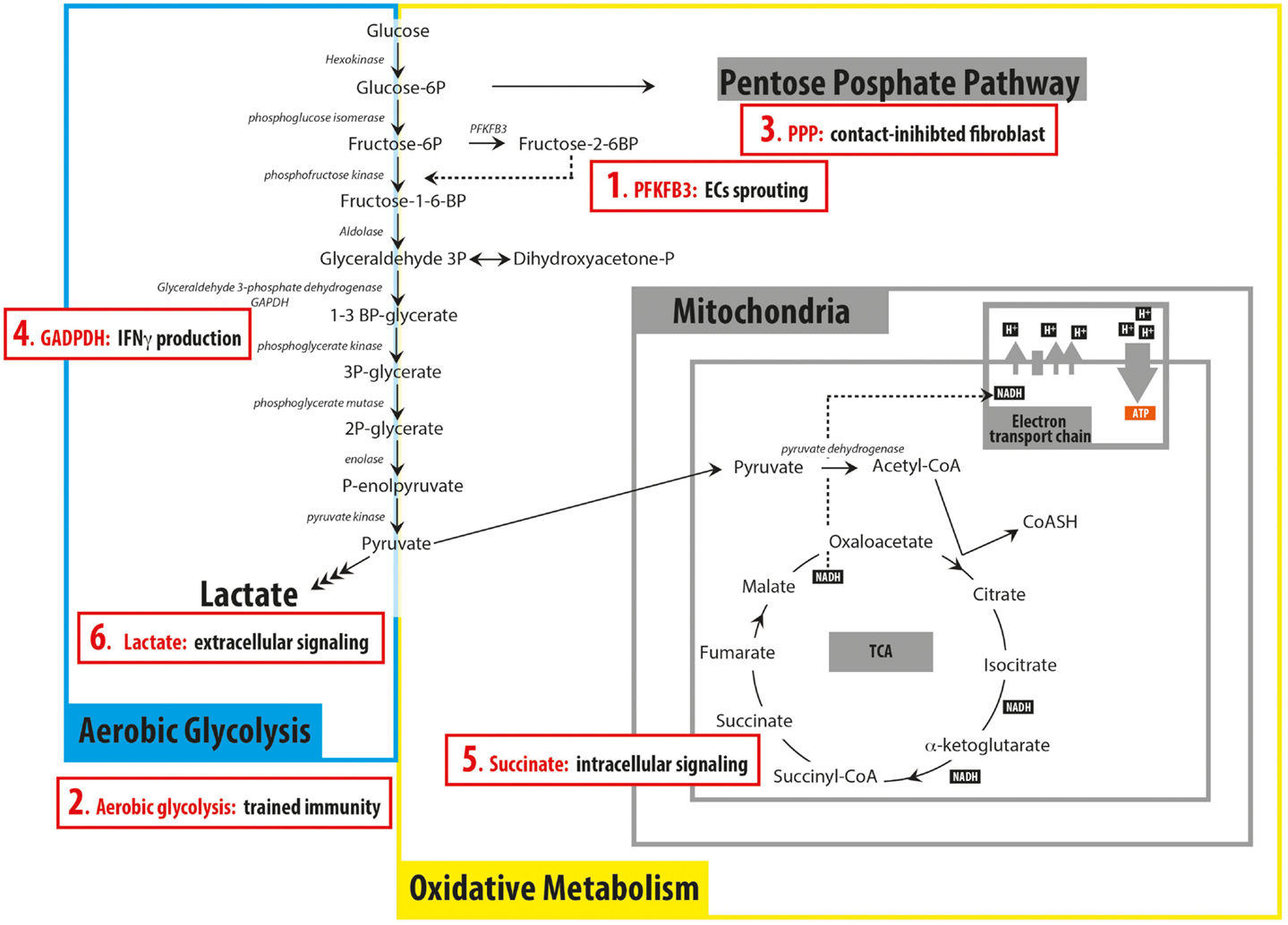